As satellites are lately my main interest and definitely the thing that my life circles around the most, there will probably be a lot of posts here about this topic. I’d love to keep them substantive and informative, but, at the same time, include as many authentic experiences as possible. The examples will be primarily based on PW-Sat2 and KRAKsat cases. Why? The answer is simple - I used to be one of the operators of the PW-Sat2 satellite, which burned in the atmosphere on 23th of February 2021, and before that, I had been involved in the KRAKsat CubeSat student project. KRAKsat was successfully launched to LEO orbit in July 2019, but we encountered so many issues that the mission ended quite quickly and violently - the satellite is now stuck in the boot loop (you can read more about it in our Lessons Learned).
What can you expect from this text? Mostly some technical (but practical) knowledge about satellite’s orbits and a few random interesting facts that were confirmed during both missions. We will discuss questions:
- what types of orbits are there
- who is responsible for providing information about a satellite’s orbit
- how long does a satellite stay in space
- how often can we communicate with a satellite
- how much time a satellite is in sunlight and how much in the shadow
- ...and few more.
So - let’s get started! :)
What is the orbit?
The trajectory of the object, gravitationally curved around another object - this is the simplest definition of the orbit you can find out there. What does it mean in practice? If there is a force (like gravity) that pulls one object in direction to the other massive object, it goes in its direction - but it doesn’t collide! If there is enough tangential velocity, it starts to go around the second object - and, tada!, we have an orbit. A good visualization of the topic is Newton’s cannonball experiment.

Fig. 1 - Newton Cannonball Experiment
If you read about the topic carefully, you will see that the Earth, Moon or other planets work in the same way - and, obviously, satellites too. If we reach a proper speed with our satellite, it will stay in the planet’s orbit for quite a long time. Orbits can be closed (elliptic) or open (parabolic/hyperbolic). We will focus on the closed orbits in this post, as it concerns almost every artificial satellite and this is our main topic for today.
But first - let’s explain some terms.
The orbital period is a concept needed to understand the satellite’s theory - it’s the time that takes an object to finish a complete orbit around the second object. The most straightforward example will be the… orbital period of the Earth orbiting the Sun - (almost) exactly 365 days. For the satellites, we are going to operate mostly in tens of minutes or hours.
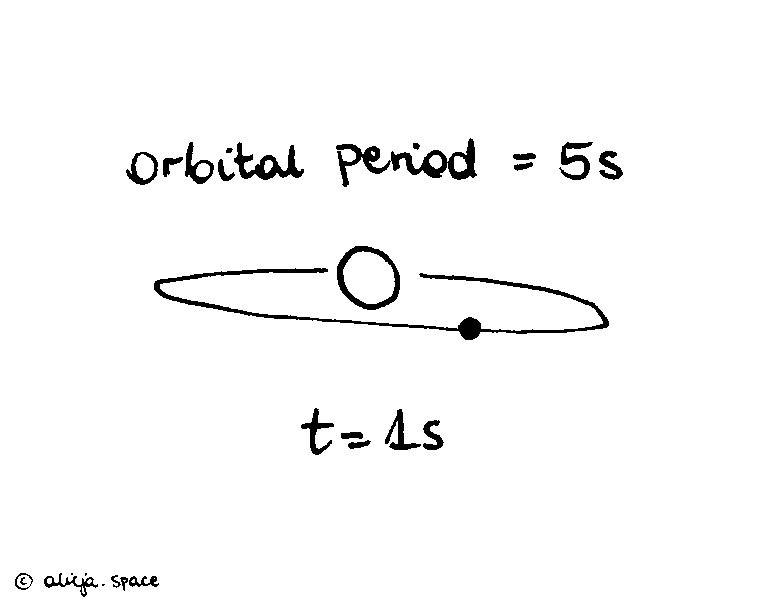
Fig. 2 - Orbital period animation (but remember, satellites are not that fast :))
We should also discuss the orbit inclination. It’s defined as an angle between a reference plane and the axis of the orbit - taking into consideration our Earth-orbiting satellite case, this parameter simply tells us what is the tilt of the orbital plane around the Earth. If the satellite was orbiting around the equator, the inclination would be 0° - and if it was circling around the poles, it would be exactly 90°.

Fig. 3 - Orbital inclination and plane
For example, let’s take the ISS orbit. It has an inclination ~50°, so visualised on the Earth in 3D, it should look like that:

Fig. 4 - ISS, orbit 3D
But what could be interesting, is its shape on the 2D projection:

Fig. 5 - ISS, orbit 2D
A similar projection will be seen for KRAKsat. Our satellite was launched from the ISS, so its orbit is really similar to the space station:

Fig. 6 - KRAKsat, orbit 2D

Fig. 7 - KRAKsat, orbit 3D
Another example would be already decayed PW-Sat2, which was put on the SSO orbit (I’ll say more about orbit’s types in the next paragraph). As you can see, the orbit was definitely closer to the poles on the 3D view:

Fig. 8 - PW-Sat2, orbit 2D

Fig. 9 - PW-Sat2, orbit 3D
And it’s also different on the 2D view. The main difference between these orbits from the operator’s perspective would be accessibility for communication from certain points on Earth, but I’ll come back to the topic later.
Conventionally, there are six orbital parameters (Keplerian elements) that define the orbit shape, size and position, but this time I won’t go into detail. Maybe in the future? :) You can always read more about it on linked websites (and there’ll also be a list of resources at the end of this article).
Types of orbits overall
But, coming back to the point - the types of orbits. If it comes to Earth’s ones, we will use the division based on the distance from the Earth’s surface. In this classification there is:
- LEO - Low Earth Orbit - from 100 to 2000 km
- MEO - Medium Earth Orbit - from 2000 km to 35786 km
- GEO - Geostationary Earth Orbit - exactly 35786 km
- HEO - High Earth Orbit - above 35786 km
I know that the one thing that strikes the most, is the weirdly precise value of the GEO orbit, but let’s start from the beginning :).
LEO is the most popular orbit if it comes to CubeSats or other small satellites created for educational, science or research purposes. Its orbital period is usually something between 90 and 120 minutes. LEO is the lowest one, which means the lowest cost for the launch, but at the same time, the shortest duration of the mission. The International Space Station is placed on the LEO orbit and that’s why periodically it has to be “moved up” a little bit (Fig. 10), as the atmospheric drag always wants to pull the station back to the surface.

Fig. 10 - ISS, orbit height
Satellites on LEO orbit are usually placed either on ISS-like orbit (~50° of inclination) or SSO, Sun Synchronized Orbit (~90° of inclination), depending on the mission goal. The velocity that is needed for the satellite not to fall is around 28,000 km/h - which is approximately 7.8 km/s! It’s even hard to imagine going so fast - one blink of an eye and your satellite has suddenly appeared on the top of Mount Everest (maybe not the best comparison, but you see the point). There's one big drawback connected to the fact that the satellite is quite low and quite fast - communication issues. It has a small field of view and communication can only be performed if the satellite is “visible” (above the horizon) for a certain place on Earth - so for LEO satellites, these communication windows are short and quite rare.
LEO orbit will be the one most important for us in terms of small satellite missions, but I’m going to briefly introduce all of the others too.
MEO, Medium Earth Orbit, is kind of a weird one. You can find mostly navigation and communication satellites there - it’s home for all GPS and Galileo projects. The orbital period of satellites placed on MEO varies between 2 to 24 hours with the most popular value 12 hours.
GEO, on the other hand, with its suspiciously precise height value, is really interesting. This height means one thing - that the satellite orbital period is 100% synchronized with the Earth’s rotational speed. So if we put a satellite above Warsaw, Poland, and the Earth will be rotating normally and the satellite will be orbiting on GEO, we’ll quickly realize that its relative position… doesn’t change! We put it somewhere and it seems to be “stuck” in this location… forever? It’s a good question and we’ll come back to it later. But for now, it’s worth mentioning that GEO is the best orbit for all communication satellites (we just point our antenna to the one precise point in the sky - and, voila!, we have some TV reception) and meteo ones, that take photos of the Earth surface and clouds - it’s so high that it can observe a significantly big area in one shot. The drawback is the latency - satellites on GEO are so far away that all communication going through them is noticeably delayed. It’s also quite expensive to put your satellite on GEO, cause it requires a lot of energy - and also some additional permits because of limits in satellite allocation in specified GEO slots. It’s a really entrancing topic and to get more into detail I recommend this presentation and this answer.
Last, but not least - HEO. Here we have some weird satellites with orbital periods longer than Earth’s rotational period, which makes them move slower than our planet rotates around its axis. Why should you even place your satellite there (besides the fact that you can)? No reasonable ideas come to my mind and I’m not the only one wondering.
My satellite is orbiting Earth… how do I know where it is now?
Okay, so you want to put your cute little CubeSat somewhere in the dark and scary space… Let’s imagine you built it, tested it, and integrated it with a deployer that was successfully put on the rocket. The rocket didn’t explode (yay!), your cube survived a launch, was deployed into its orbit directly from the deployer on the rocket or from the special ISS module - and it’s now completely alone drifting in the void, a few hundred kilometers above your head… How do we know where the satellite is at this exact moment? Who is tracking it and providing the information about its orbit? Where and when should we wave our antennas to communicate with it?

Fig. 11 - Where is the satellite?
The answer is: 18SPCS.
What does this weird abbreviation hide and why does it sound like… military thingy? The answer is simple - because it is. 18th Space Control Squadron is an American army space surveillance unit responsible for tracking all the objects on orbit, from satellites to debris, and predicting their possible collisions. Information is publicly shared and available on the Space-Track website. It’s known to use observations from all available sources: optical, radar, radio and visual. The final computation about a certain satellite is published in the form of so-called Two-Line Element, TLE (but to be honest - the funny issue with a TLE is the fact that in reality, it has three lines, one for the satellite name and two for the orbital parameters). Every satellite has also a unique NORAD identifier assigned (NORAD is another American army unit that is responsible for space-related stuff in the USA).

Fig. 12 - PW-Sat2, TLE
From the satellite operator’s perspective, the most important thing is the already mentioned TLE (which history dates back to the 50's!). In the form of a few mysterious numbers, it includes mean motions, inclination, eccentricity and other orbital parameters that are necessary to compute the satellite orbit and predict where it will be flying at a certain time. For the so-called orbit propagation, we can use SGP4 simplified perturbation models’ based algorithms implementations (e.g. python one) or any existing software like GPredict/Orbitron (for desktop) or my web project tracksat.space. The best source for the actual TLE (as new ones are generated once a day/few days) can be the official Space-Track or not-official Celestrak (which was founded by one person - Dr T.S. Kelso). Celestrak is easier to access - all TLEs are available in form of simple *.txt files.

Fig. 13 - KRAKsat, current position computed from TLE
How long will my satellite be in space?
The answer is, of course: it depends. It’s quite intuitive that the orbit height and type is the most important factor when it comes to the mission duration - if we put our satellite on ~400km ISS-like orbit it will surely fall quicker than a satellite on the SSO orbit (~600km). But let’s take into consideration some real examples. With KRAKsat we speculated that the mission will last about 1-2 years. It was launched from the ISS on the 3rd of July 2019, together with another polish satellite - Światowid. KRAKsat was CubeSat in size 1U, which means a cube with dimensions 10x10x10cm, while Światowid was 2U (~20x10x10cm) with quite big foldable solar panels - so the final shapes of both satellites were totally different. These differences led to the current situation - the KRAKsat satellite is still happily rotating (and rebooting) around the Earth while Światowid… deorbited two weeks ago (around 16th of March 2021). Predictions (created by Tomasz Martyniak, many thanks!) show that KRAKsat will probably decay in December 2021, so it will spend 9-10 months more in orbit than Światowid, released the same day.

Fig. 14 - KRAKsat, deorbit prediction
KRAKsat example shows how important is the size and shape of the satellite when it comes to deorbit, but an even more figurative one would be the case of PW-Sat2. PW-Sat2 mission was to… deorbit as quickly as possible. It was launched into SSO orbit, ~590km above the Earth, on 3rd of December 2018 and after 27 days its deorbit sail was released. It was a 4m2 flat mylar foil attached to the 2U CubeSat serving as the experimental deorbit kit. Satellites released at these heights usually stay in orbit for even 20-30 years while their missions often end earlier and they are no longer needed there. PW-Sat2 reentry was predicted to happen after around two years - and the prediction turned out to be quite precise because it burned in the atmosphere in February 2021, exactly 2 years and 3 months after the launch.
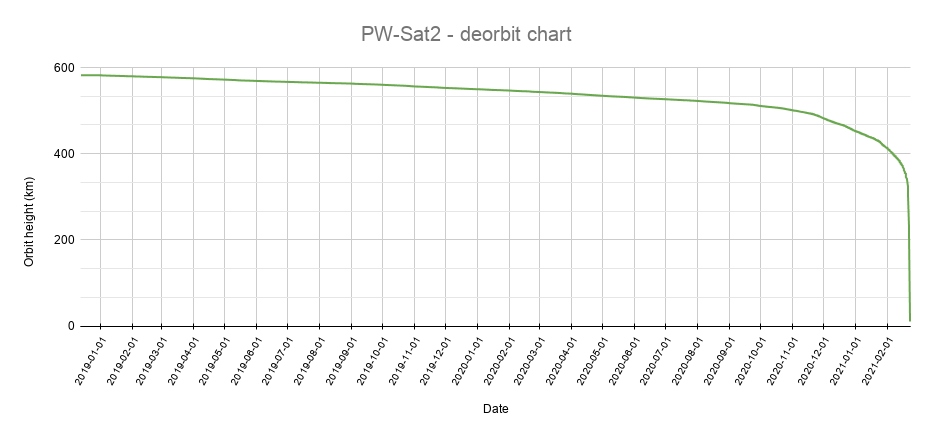
Fig. 15 - PW-Sat2, deorbit chart
Two other polish satellites which are part of the international BRITE constellation, Lem and Heweliusz, were both launched into SSO orbit too, sequentially in 2013 and 2014 - and they are still in orbit and operative! With mean height a little bit above 600km, they are forecasted to spend even 40-50 years in space overall. I even tried to compute their lifetime prediction in GMAT software, but it was too much for my computer :). But there's one thing I can confirm - in 2045, Lem will still happily fly above our heads.
And what happens after all these years? It again - depends on the orbit. As it was mentioned before, satellites on LEO come closer to the Earth, little by little (exactly like on KRAKsat or PW-Sat2 altitude charts), and finally, they enter the atmosphere and burn probably somewhere around 150km above our planet surface. Big spacecraft need to have a planned and controlled reentry, usually somewhere above the ocean to make it safe, but CubeSats and other small satellites are entirely destroyed during the process. If you were lucky, you would see a short flare in the sky, just like with a meteorite - but the chances are extremely low because it would be quite hard to predict where the reentry happens. The last days of the satellite's life are pretty interesting and it’s not so obvious to get its position right (even TLE are outdated after a few hours), but it’s probably a topic for another post.
Coming back to the end of satellites’ life - what happens to satellites on GEO? We were discussing this weird orbit earlier and its insane distance from the Earth’s surface (exactly 35786 kilometers). It’s easy to predict that they are probably not going to come back - so, what happens? There is this thing called a graveyard orbit. It is located around 300km above the GEO and serves as a disposal place, where satellites can be moved at the end of their operational life to reduce the possibility of potential collision or interference with working ones. All geostationary satellites are equipped with some kind of propulsion to be able to keep their position during their missions - transferring them to the graveyard should take a similar amount of the fuel that could be used for a few months of stationkeeping. Not every satellite succeeds with this maneuver, but every single one that doesn’t is a potential threat for ongoing missions and occupies a precious slot on the stuffed GEO orbit.
Because of all these facts, the satellite debris issue has been such a hot topic for quite a long time. Not only loads of new satellites that are placed in orbit every year are a problem (every satellite owner has to assure that it’ll be gone in a few years), but mostly the satellites that are already there. Many old and unused devices are still drifting around the Earth (e.g. CALSPHERE 1, which has been in orbit since 1964!), sometimes causing problems - like this meteo one.
But we’re not here to discuss debris (at least - not today!), so let’s come back to our cute imaginary CubeSat currently orbiting Earth. Thanks to 18SPCS and SpaceTrack we got its TLE and, after calculating the position in one of the mentioned software, we know that it will fly above us, let’s say, at 11 a.m. So, we point our antennas to the horizon, as the computed polar plot shows it passes from east to west, but… what should we prepare for? Quick two-minutes pass? Visibility for half an hour? How many commands can we expect to send? These doubts lead to the important question...
How long and how often can I communicate with the satellite?

Fig. 16 - Communication with satellite on LEO orbit
Are you already bored with the answer “it depends”? If yes, I have some sad news… But I promised that we’re going to talk with real-life examples, so - let’s start from KRAKsat!

Fig. 17 - KRAKsat passes over Warsaw, LEO, ISS-like orbit (7.04.2021)
As you can see in the above screenshot, we are going to have six passes tommorrow (7.04.2021) and they are going to last 8 to 10 minutes on average. Elevation varies from a few degrees to 80°, and as you can probably imagine, the communication will be much better during the higher ones. The gap between passes is also important - they appear in a series with 90-minutes intervals because that’s the orbital period for KRAKsat. If we make a calculation for the next day…

Fig. 18 - KRAKsat passes over Warsaw, LEO, ISS-like orbit (8.04.2021)
...we can see that the first pass moved about an hour back - the series starts a little bit earlier now. If we compute more passes, we’ll see that the pattern is 100% repeatable and the passes series is going to move backward in time every day.
What does it mean in practice? Communication sessions will have to be performed sometimes in the evening, then in the morning, and periodically even in the middle of the night. From the satellite operator’s perspective, it could be inconvenient and tiring, as there is no way to apply constant and stable work hours.
If we look into the orbits in terms of the comfort of the person responsible for communication, we should probably consider putting the satellite on the SSO. Sun-synchronized orbit’s characteristics lead to the fact that the object on it is visible from the chosen place on Earth more or less at the same time every day.

Fig. 19 - Heweliusz, passes over Warsaw (8.04.2021)

Fig. 20 - Heweliusz, passes over Warsaw (9.04.2021)
If we compare a few days of passes for Heweliusz, we see that hours of passes are recurring - we can expect around three 9-13 minutes passes around noon and three 9-13 minutes passes around midnight. Predictability leads not only to the operator's settled schedule (and good mood), but also allows the satellite to fulfill some specific mission’s goals - mostly connected to Earth observation. To simplify the concept - let’s assume we want to take photographs of Poland’s capital from orbit every day. If we put our satellite on ISS-like orbit, in half of the cases we will get photos from the day (good!), but in half of the cases… from the middle of the night (not really usable). SSO would be the most convenient orbit here because it’d assure that the satellite will take a photo more or less exactly on the same hour every day.
Of course in the above case, we could probably also consider GEO - “glueing” a satellite above Warsaw could sound like the best scenario ever. And this orbit is often used for these purposes, mostly for meteo satellites. But as I mentioned earlier - it’s harder to get access to a free slot on GEO and placing a satellite there requires remarkably bigger funding - rather inaccessible for CubeSats. But for this post completeness let’s consider GEO communication sessions. Being an operator here could be quite boring because we have two possible scenarios - satellite can be visible all the time or… invisible all the time.

Fig. 21 - Communication with satellite on GEO orbit
It’s quite intuitive - if we place the spacecraft above Sydney, it couldn’t be visible from, for example, Warsaw - but Australians would see it all the time. Easy? Easy. So - let’s put a little light on the next interesting topic...
How much time a satellite is in sunlight and how much in shadow?
And this is a topic this article was meant to be about! To be honest, I just wanted to write about a small discovery I made during the KRAKsat mission about the sun synchronization on the LEO, but when I sat down to write this post, it started growing, growing, and growing… But we are here - last, but not least - and we can finally discuss this extremely exciting topic.

Fig. 22 - Umbra, penumbra & the light
Why is this even important? Imagine the satellite drifting in the orbit. Its only power source is usually solar panels, so the amount of solar energy that can be got to charge the batteries it’s extremely significant in terms of any space mission. More time in the sun means more energy and the energy budget should be thoroughly matched to the orbit conditions (including the sun and shadow time).
One thing that is widely known - how much time a satellite spends in umbra (shadow) and penumbra (almost shadow) is determined by the orbit height and inclination. When a spacecraft is orbiting Earth, for a part of the orbit it is located between the Earth and the Sun (happily using the sunlight to charge the batteries) and for the next part - behind the Earth (drifting in the dark). The duration of these two positions varies a bit between seasons because the position of the Earth in reference to the Sun is changing.
Another fact that is copied across many materials is the ratio between time in the shadow and time in the sun. For ISS-like orbit (like KRAKsat) in one day it should be about 9 hours (35-40%) in the dark and 15 hours in the light (60-65%). And this assumption is true… almost.
KRAKsat satellite - time in sun&shadow (LEO)
If we create a chart for KRAKsat with a calculation of the time that was spent in the umbra and penumbra for every single day of its mission we’ll quickly realize that this assumption was correct only partially. Indeed, the average time for the whole mission is something about 9 hours of darkness a day, but the real values are quite diverse! Let’s have a look at Fig. 21:

Fig. 23 - KRAKsat, eclipse time
What can be shocking at first are these deep gaps, when a time in shadow daily is oscillating around 0 hours. How can it happen? Does it mean that the satellite can be in the constant light for a day or a few in a row? In short words: Yes. And the answer “how” is trickier than it may seem - and it includes the terminator. Of course not the Schwarzenegger one, but the Earth day/night one. There are some days, and it can happen around 2-4 times in the year when the orbit “synchronizes” with the terminator and the sun for the satellite doesn’t set for some time (or it does only for a while). Sounds crazy, doesn’t it?

Fig. 24 - Terminator synchronization on LEO
Trying to explain that from the scientific point of view, we would quickly drown in orbital mechanics, but I’ll try to do it smoothly. The key here is the so-called beta angle. It is defined as an angle between the orbital plane of the object and the sun. Having a 90-degree beta angle would mean a satellite being constantly in the sun, while 0-degree will have it in a 50/50 ratio. But don’t mix up beta angle with the inclination! The inclination is fixed, while the beta angle is not. For KRAKsat (or ISS) the inclination is about 51°, while beta angle changes when the Earth-Sun arrangement alters its tilt through the year, reaching it maximum at around 20°. It means that the maximum 70° and minimum -70° beta angle is possible in this scenario and when it happens, the full-sun period starts. Summarizing: the correlation between the satellite orbit around the Earth and the Earth orbit around the Sun causes this fascinating behavior.
If you read more about the KRAKsat mission, you will probably notice two interesting facts that can be somehow related to the sunlight. Out CubeSat was put on its orbit on the 3rd of July and it remained silent for 2 weeks. It suddenly turned itself on on 16th of July, when it was recorded by a radioamateur from SatNOGS and we got to know it’s alive and well. If you have a look into the eclipse chart on Fig. 21, you’ll realize that around this date a sun period has started! Of course, it could be only a coincidence, but my theory includes a jammed killer switch that lets go after being warmed up by constant sunlight… It might have been like that or not - we would never know, but the temperature charts show a bit higher values for this period.

Fig. 25 - KRAKsat, temperature charts
The second thing is the constant reboots. KRAKsat is in the boot loop and it’s restarting all the time with quite a regular interval. But… it isn’t always regular and you have probably already guessed that it’s connected to the light periods. Below you can find a reboot frequency chart:
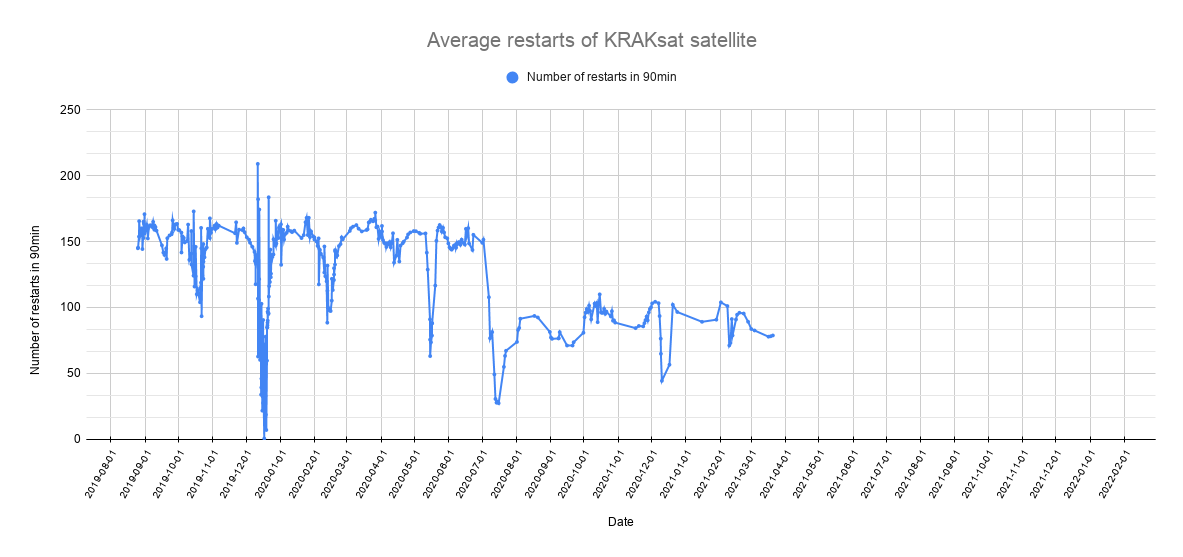
Fig. 26 - KRAKsat average restarts in 90min
...and a reboot frequency chart correlated with the eclipse chart:

Fig. 27 - KRAKsat eclipse & restarts correlation
Do you see it? For the first look, it can be a bit unintuitive, because it restarts less in the sunny periods, but if you think about it more it does make sense. When KRAKsat is constantly in the sun, it gets enough energy to survive the restart, so the intervals between reboots are longer - it’s alive for a while! Unfortunately, it’s not enough power to do anything meaningful (every attempt to communicate causes only a quick switch off), but it’s interesting to observe these repetitive patterns.
Just out of curiosity, let’s look at two interesting data points on this chart:

Fig. 28 - KRAKsat eclipse & restarts correlation with interesting data points
And if you ask what happened in July 2020 (marked as Y on Fig. 26), when the frequency changed by itself, dropping by half - I know exactly as much as you. It just did and we were speculating that maybe something changed electronically/mechanically with one solar panel - but as it’s on the orbit, we will never know the truth. The reboot frequency is the only data I can get from the satellite, but if you have any idea what this number can be used for - don’t hesitate to write!
But the second marked place is more interesting - X. We can see that the shadow time was a little bit longer that day, something about one hour more than usual. So if we google “June 21st, 2020”, the… solar eclipse details will appear! It seems that KRAKsat saw the partial eclipse from the orbit (it was quite a surprising fact for me, but the computations leave no doubt that it happened.
The last intriguing chart will be one involving Światowid. I mentioned that the sunny periods were slightly visible on KRAKsat temperature charts, however it was hard to tell if it means anything because we only have a few valid data points (it was working properly only for ~2 weeks). But for Światowid we have more data, so let's have a look:

Fig. 29 - Światowid temperatures and eclipse correlation
...and yes, it's there! When the eclipse time chart is dropping near 0 and only-sun period starts, we can see a sudden temperature change.
PW-Sat2 satellite - time in sun&shadow (SSO)
And what about PW-Sat2? As I mentioned a few times, it was put on SSO, about 590km above the Earth's surface with ~97° of inclination. By the way, why is this value bigger than 90°? The orbit was slightly retrograde, which meant the satellite’s rotation direction was opposite to the Earth rotation direction - inclination between 90 and 180 degrees indicates this type of movement.
So let’s see the eclipse chart:

Fig. 30 - PW-Sat2 eclipse time
The first thing that strikes is the lack of the zero-shadow periods. For this kind of orbit, the synchronization with a terminator is not possible (beta angles again), so the light/umbra ratio is quite constant - eclipse time is around 8-9 hours daily (35%), with 15-16 hours (65%) of sunlight. There are also sun eclipses visible, just like with KRAKsat - but PW-Sat2 saw as many as 5 of them! Maybe it’s not the best example for the SSO, because, as I wrote earlier, its goal was to deorbit and the opened sail clearly changed the orbit properties and the velocity of the satellite, but it’s also interesting to see how the eclipse time changed over time for this kind of spacecraft.
Satellites orbits - a summary
To summarize all the things that we discussed, there are few facts that I was trying to make a point about:
- artificial satellites can be put on varied orbits with different heights and inclination - but the most popular one for small satellites is LEO
- satellites on LEO can stay in orbit for such a short period like one year or even for 20-or-more years (depends on the height and, what’s even more important, on the satellite’s size and shape)
- communication session for an average CubeSat lasts about 10 minutes - a horribly long time when you are waiting for a bus, but fearfully short if you want to perform your mission
- time spent by a satellite in sun and shadow varies not only with its inclination, but also with Earth tilt and can be disrupted by terminator synchronization. Also, Sun eclipses can be “visible” for the satellite
If you made this to this point - thank you, it means a lot to me :).
Big thanks!
The greatest high-five here belongs to Tomasz Martyniak, whose help was invaluable - mostly with computing all the data for charts and understanding the nooks and crannies of GMAT software. Tomasz was also the first person from our team who originally realized that ISS orbit is not that evenly illuminated, which helped me to interpret KRAKsat data better - so, thank you!
Sources
Below you can find a list of sources that were used while writing this article. Probably I missed some materials because the research took me quite a long time and some of them may have been lost in the browser history void, but if you want to explore the topic more, these links should be valuable and worth reading either way :)
Have fun:
- https://www.nasa.gov/smallsat-institute/sst-soa-2020/identification-and-tracking-systems
- https://www.nasa.gov/sites/default/files/atoms/files/nasa_csli_cubesat_101_508.pdf
- https://phys.org/news/2017-04-satellites-die.html
- https://spectrum.ieee.org/tech-talk/aerospace/satellites/zombie-satellites-return-from-the-graveyard
- https://celestrak.com/
- https://www.space-track.org/documentation#/faq
- https://static1.squarespace.com/static/53d7dcdce4b07a1cdbbc08a4/t/5d7680c0868cde02a3e2cf60/1568047299430/18+SPCS+Brief+for+PocketQube+Workshop+Sep+2019.pdf
- https://swfound.org/media/205965/mckissock_cubesat_recommendations_aug2017.pdf
- https://www.spacecom.mil/News/Article-Display/Article/2360595/18-spcs-now-predicts-debris-on-debris-collisions-in-space-enhancing-space-domai/
- http://www.esa.int/Enabling_Support/Operations/Space_debris_mitigation_the_case_for_a_code_of_conduct
- https://blogs.brown.edu/umbricht/2018/10/09/satellite-orbit-seasons/
- https://space.stackexchange.com/questions/4686/how-often-does-the-iss-orbit-align-with-the-day-night-terminator
- https://space.stackexchange.com/questions/27361/what-does-the-iss-ground-track-look-like-during-periods-of-high-solar-beta
All graphics and charts are of my authorship, while screenshots come from my web app tracksat.space :).
