The day has come. KRAKsat burned during a reentry yesterday. This cute but unsuccessful CubeSat, which I had the privilege to work on, started its space adventure on the 17th of April 2019 with the launch of the Antares rocket NG-11 mission. After the flight, it waited almost 3 months to be released from the International Space Station, and when the deployment finally took place (3rd July), it turned out that the satellite was… silent.
Five years ago I’d have never imagined that I'd ever work on a device that will (literally!) orbit around the Earth. Now it’s hard to imagine doing anything else. But with my first space child reentry, an era has just ended and it felt right to write down some things. I would like to tell you a few interesting facts and show a handful of statistics about satellites’ deorbit overall, at the same time paying tribute to the small cube that has just burned during the tragic yet inevitable attempt to come back. I hope it'll be engaging for you – so let’s start with a quick history recap.
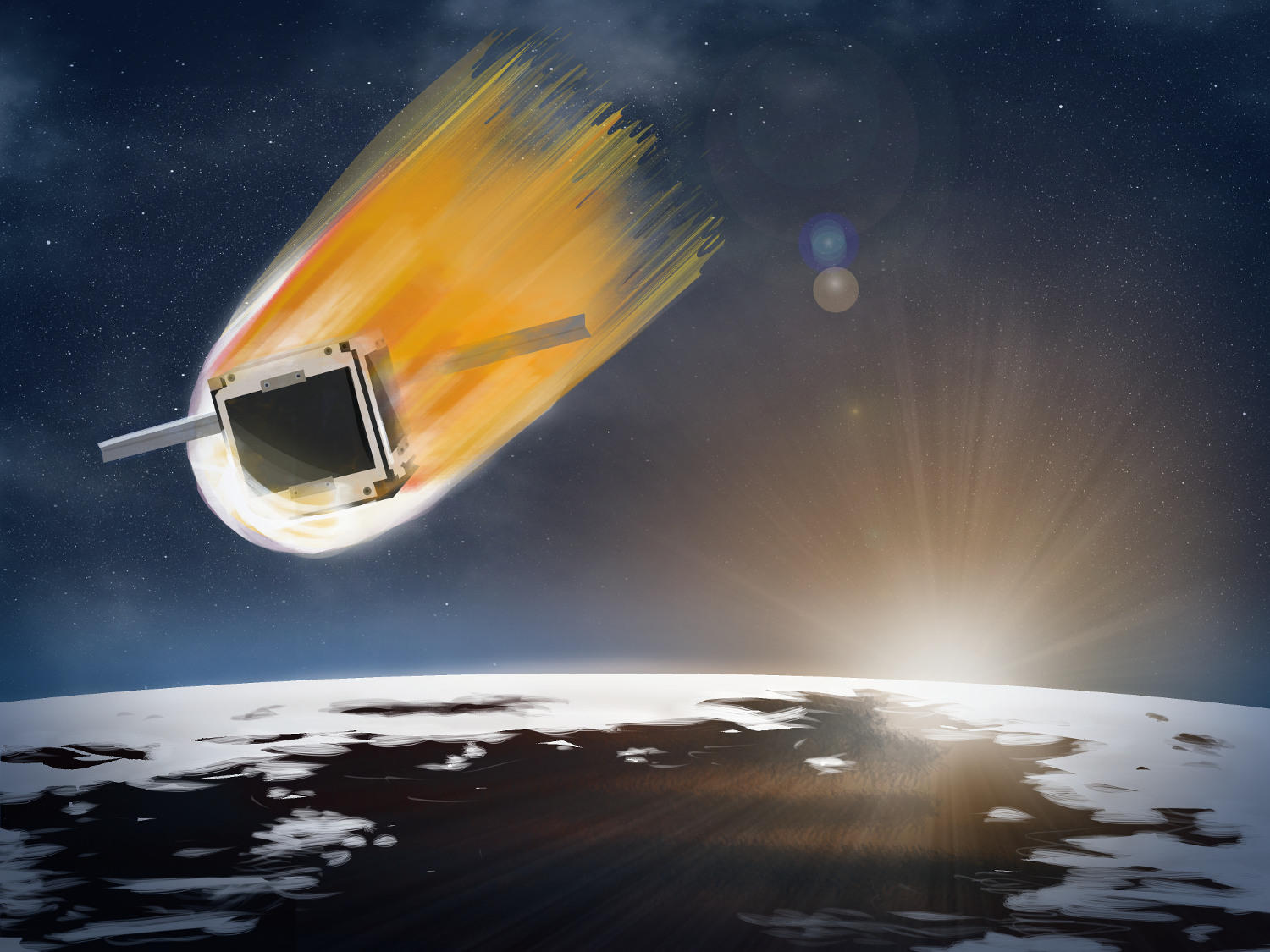
Fig. 1 - Did I draw a burning KRAKsat? Yes, I did. Let's call this overcoming trauma...
Short story of a restarting cube
After the deployment from the ISS, our cube didn’t say its first words. Dead on arrival? Have we just increased the satellite's infant mortality rates? The days passed one by one and we gradually lost hope. Światowid, released together with KRAKsat, broadcasted as it should, but our cube remained silent. Suddenly, the big surprise came – on July 17th, while I was browsing the SatNOGS database, with amateur radio observations from all over the world, I came across something breathtaking. KRAKsat’s beacon! The cube was transmitting as if nothing had happened.
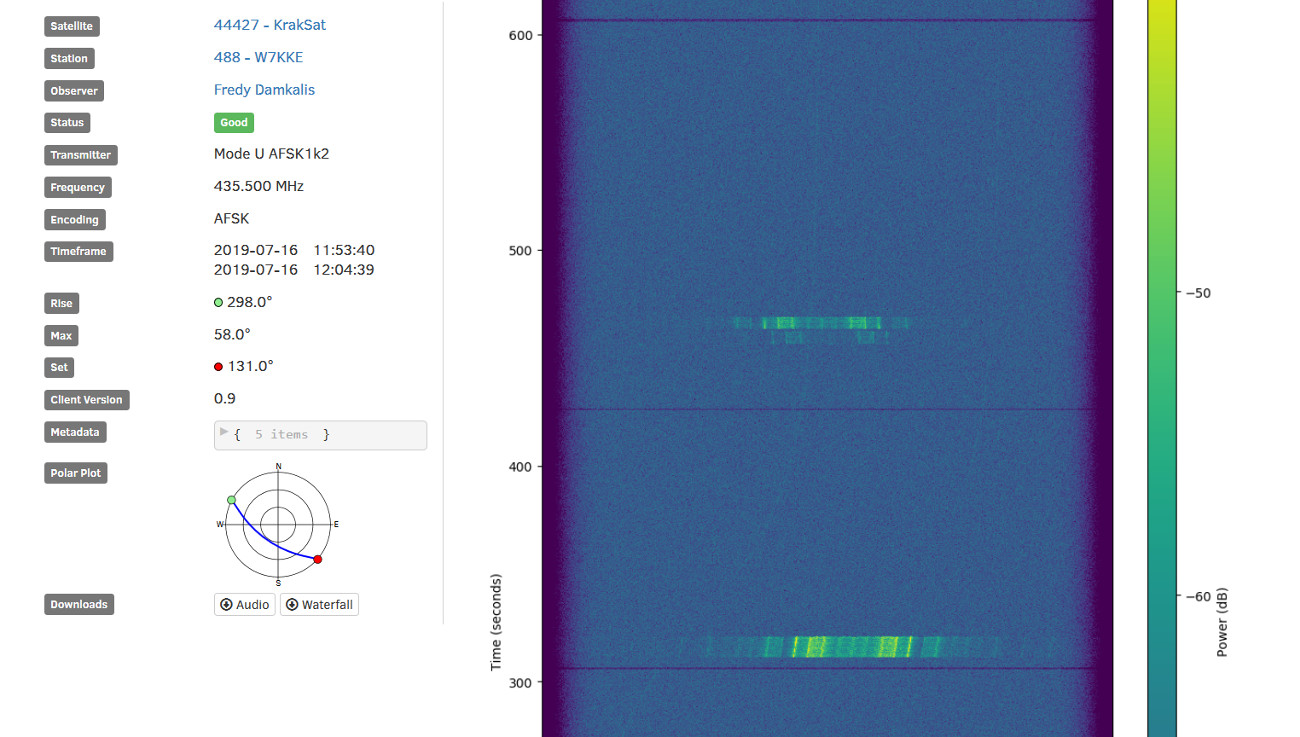
Fig. 2 - First KRAKsat beacon (signal at the top, around 450s)
Unfortunately what occured later was a cumulation of errors and mistakes – effects of these committed before launch as well as during the operations phase. KRAKsat was working, temporarily, but its battery value was decreasing quickly and irreversibly. There wasn’t enough time to perform the experiment, and, as we found out in panic, no possibility to stop discharging. On 30th July, when it discharged below the operative level, a new bug in the platform's EPS was discovered – lack of battery voltage hysteresis – as a result of which the satellite fell into a reboot loop. The cube had been restarting constantly (in the sun) all the time until the moment of reentry. It burned in the atmosphere yesterday (18th of January 2022), at night, with the counter reaching 1713728 reboots in the last decoded message.
There was an attempt made to try to rescue KRAKsat and, at least, get some data from the experiment already saved in its memory. As it was behaving differently in the whole-sun-period (I described this phenomenon in detail in the article about orbits), the decision was made to try to switch transmission off before the battery discharges, wait some time (hoping it would charge a bit), and download data. Unfortunately, it turned out that KRAKsat cannot be charged above a reasonable level, even when it’s constantly in the sunlight and the transmission is turned off. However, we managed to prove that the memory doesn’t contain any meaningful data that would make sense to fight for. The only option to know the results of the ferrofluid experiment would be to completely clean the flash memory, and perform the experiment again – and at its power level, it would be completely impossible (to read more about why read our lessons learned). Pity.
That was KRAKsat’s memoir in a very few words – in fact, its story was a sinusoid of extreme feelings. Happiness and satisfaction after it was integrated with a platform, anxiety, and stress about the rocket launch, sorrow with a glimmer of hope when it didn’t transmit after deployment… And finally, an emotional rollercoaster when the first beacon was spotted and the real mission began (with an endlessly growing pile of problems). To be honest, I’m pretty sure that it made me addicted to adrenaline shots, as I miss these crazy months of extremely intensive work. 10/10, would do it again.
🎵 I'm passing over you like a satellite, so catch me if I fall 🎵
Okay, let’s talk about reentries.
There is this song by Rise Against that I like (not only because of its title!) but this one verse always makes me laugh. I'm passing over you like a satellite, so catch me if I fall? This does not sound safe. Nor likely at all. Fortunately, so far no one has yet been killed by deorbiting space debris but in fact, there was one person in history that was struck by a part from the falling rocket (in 1997). Taking into consideration how the reentry process looks and how large and tough the object has to be to survive the fall – nope, I wouldn’t like to try to catch it, thanks.
While writing this article, I came across a database that includes all the debris that made its way back to the Earth (or was supposed to do so, according to its size and weight). And it’s in .csv format – nice! There are quite many entries in the database including some sizable payloads, worn rocket segments, and random debris (funny category). According to this list, in the first half of January 2022, there were already almost 20 parts that came back to us from space. In 2021 150 objects made their reentry while in 2020 – 92. In general, there are 1322 objects in the dataset for now but I can imagine that it’s constantly growing.
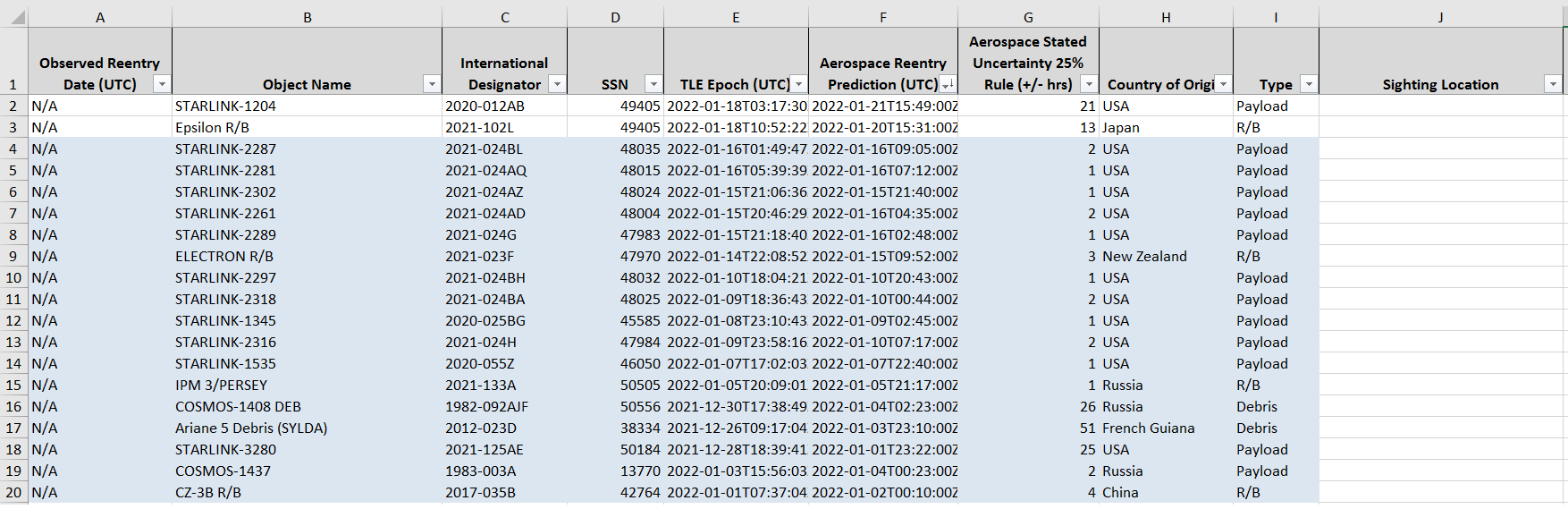
Fig. 3 - Big objects that have already reentered in 2022. So many Starlinks!
If you look at this file, you should quickly realize that there is no information about smaller spacecraft and their fate. It appears that the authors didn’t pay a lot of attention to CubeSats and other miniature satellites, which is a little bit disappointing. They did, however, focus on some random stuff like… tutorials on how to identify if the object you see falling is a meteorite or a human-made spacecraft reentry. And you can report seeing one, too! So maybe Rise Against still has a chance…
How many CubeSats have fallen until now?
We can try to extract the information about smaller satellites’ comebacks from other sources, e.g. Nanosats Database or Swartwout database. The first one is quite a pain to analyze as data is embedded into an online table (that cannot be downloaded as *.csv). Additionally, deorbit is marked not in a separate column but is a plain text annotation in the random field, so I’m not 100% sure that I found them all. But let’s suppose that I had a little bit of luck – therefore the table (filtered by CubeSats, ~1660 records) says that in 2021 there were 38 reentries, or as it should be highlighted, 38 complete burns in the atmosphere. Overall, there are 333 CubeSats’ deorbits in the whole database since 2009. Note: these statistics don’t include constellations.
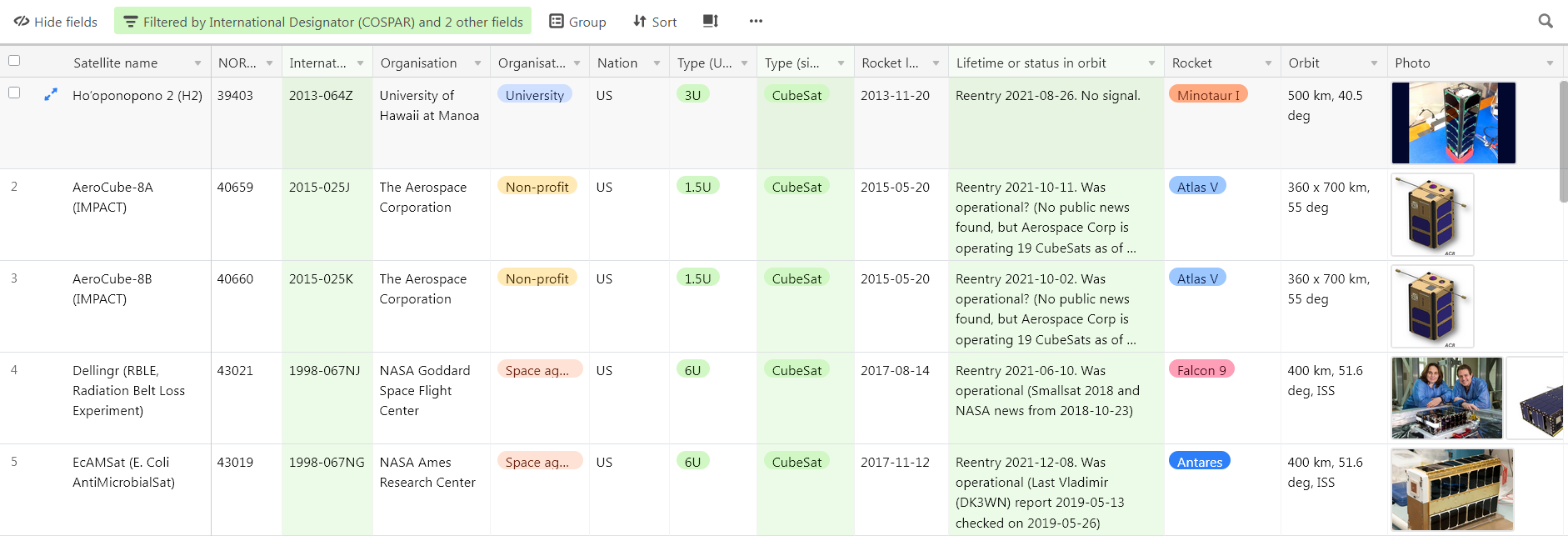
Fig. 4 - Screenshot from the Nanosats Database - why does the reentry not have its column?
Swartwout's database stores data in a little bit different way (that is also quite hard to analyze automatically…) but there is a small interesting table available that shows how many satellites were already decayed. Unfortunately, I couldn’t find information when exactly it was updated but data looks quite legit – it says that more than 440 CubeSats from 1532 launched have already decayed.
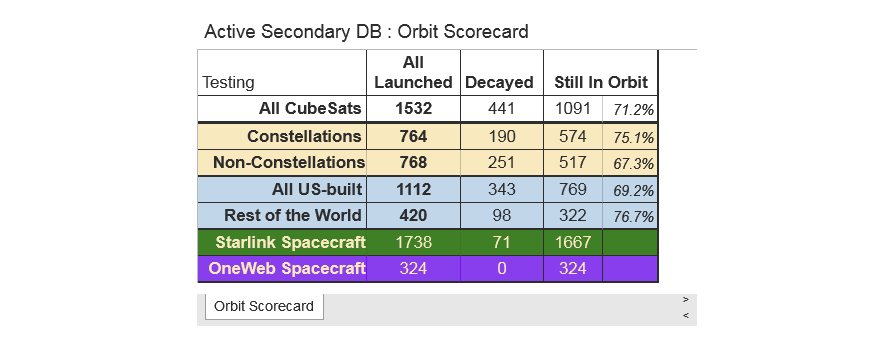
Fig. 5 - A table from the Swartwout database
The table (Fig. 5) adds Starlink to the image, pointing out that there are >1700 of them placed in orbit with already a few dozen decayed. It is interesting to find Starlink in this diagram though, as they are quite far away from the nanosat category (weighing ~260 kg each), and definitely not CubeSats. But if it comes to preparing statistics about orbit occupation or satellites’ deorbitation, it’s good to include them – they are planned only for a few years working in space, so we should expect hundreds of their reentries in the not-so-far-away future.
Look at the numbers from both databases once again, do you see that something is wrong? They differ quite significantly, as there are fewer CubeSat recorded in the Swartwout entry set but the number of decayed objects is higher. What can I say? Analysis of satellites’ deployments is not very complicated – in every rocket launch there is almost always a full list of its payload available somewhere. Problems begin when missions start. Sometimes there are some updates on social media/official channels about the satellite’s fate or someone is nice enough to write a scientific paper but there are no rules or regulations that require informing the public about what happened to the device – is it operative, is it going to deorbit, etc. You can of course try to track TLEs and automate detecting if any satellite performs a reentry but I don’t know if this method is used by databases’ maintainers. But maybe it should be? Hush brain, it doesn't mean I should make my own database, it doesn't mean that, nooooOOooOoo...
What makes satellites fall?
As you probably already know (maybe from my first article about this topic?) orbit is a trajectory of an object curved gravitationally around another object. To be able to fly steadily around the Earth our satellite has to gain a proper orbital speed. The higher we place it, the lower velocity it needs to remain there. You can see the speed’s comparison in Fig. 6 – KRAKsat placed at ~400 km orbit had significantly bigger velocity than PW-Sat2 at 590 km.
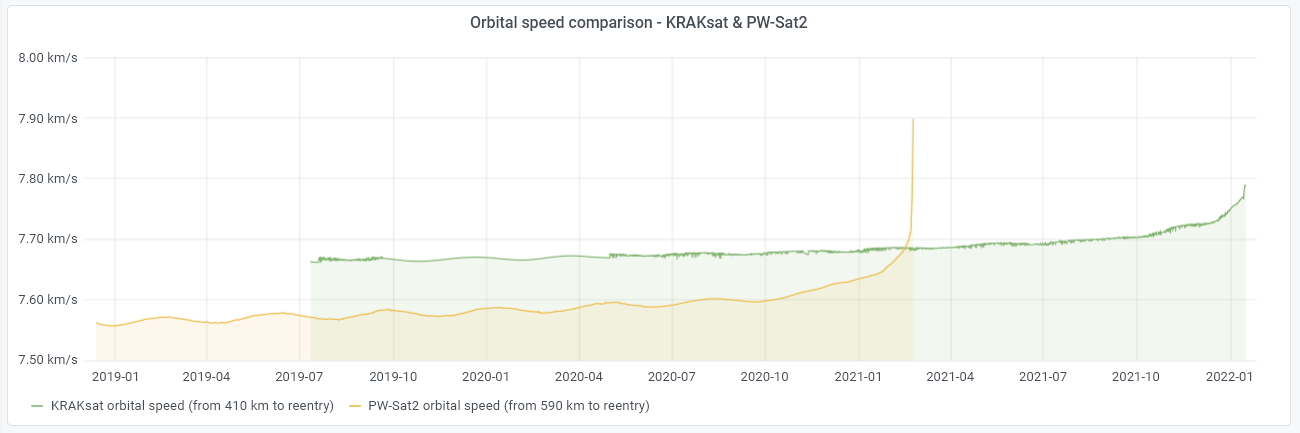
Fig. 6 - KRAKsat & PW-Sat2 orbital speed - and who is the fastest space child?
❯ Note: If you haven't read my previous article about orbits yet, I strongly recommend doing so – I’m going to splurge on many abbreviations and concepts that were described thoroughly in it and I don't want to repeat all explanations here :).
Consider a satellite located in a perfectly circular orbit – it should stay on it forever because of its orbital speed, and the gravitational force that keeps the circular motion going.
But it does not.
Why? There are some forces that transfer a satellite's energy from the orbital motion. In the lower orbits, the most significant of them is the atmospheric drag. It can be defined as constant gas molecules collisions with the object in space. The lower the satellite is, the more molecules “attack” it, and the bigger drag is affecting its journey – this is one of the reasons why the spacecraft falls quicker and quicker when the height is smaller. Another thing that has an effect on satellites’ paths is perturbing acceleration from the Sun and Moon. Both are quite far away but their influence also should be included while performing some calculations. According to Reference 1, solar radiation pressure is also an important factor – this small but not negligible force arises from the absorption of photos by satellite’s surface area.
The drag, mentioned earlier, is severely affected by solar activities. There is a whole branch of specialization focused on the impact that solar flux has on satellite lifetime – its modeling and predictions that allow foreseeing how long the object is going to stay in its orbit.
The solar activity is known to have a significant influence on the upper atmosphere and near Earth environment. When solar activity is high, ultraviolet radiation from the Sun heats and expands the Earth's upper atmosphere which in turn, increases the atmospheric drag and orbital decay rate of the satellites – says abstract of Reference 5. It means, in short, that intensive solar activities cause quicker spacecraft falling and reduced solar activities lead to prolonged time in orbit.
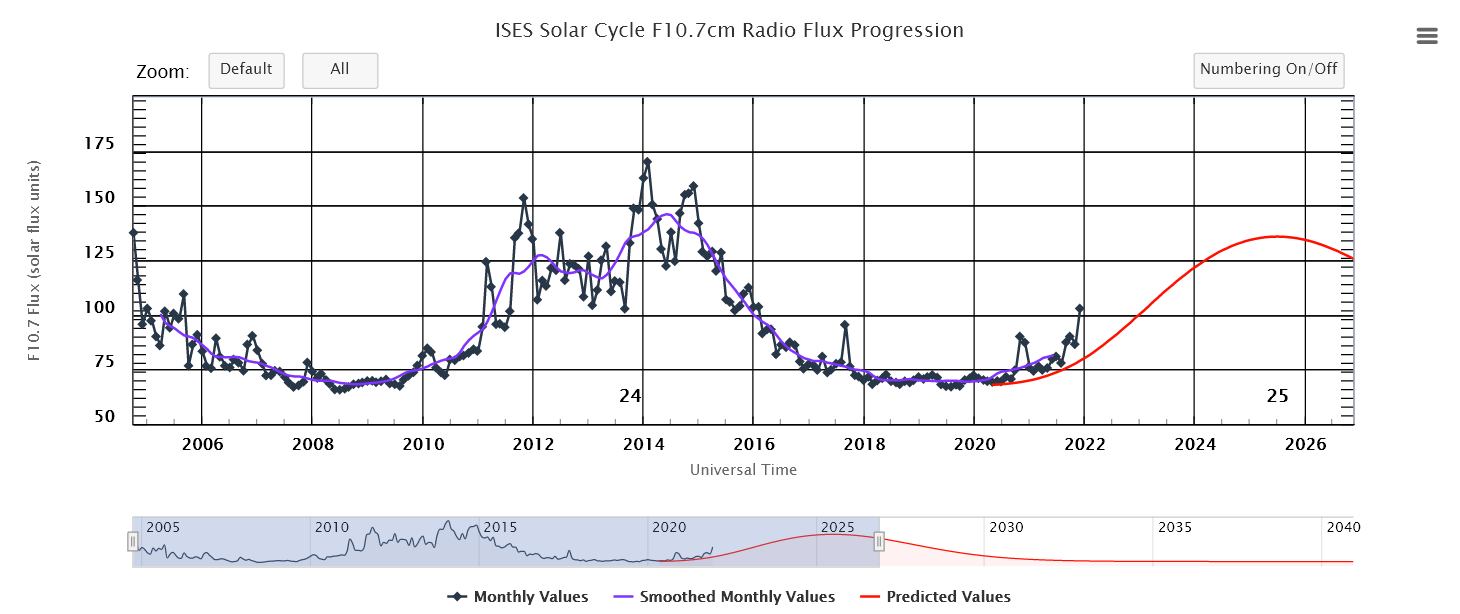
Fig. 7 - Solar activity with a simple prediction for the forthcoming months
Source: https://www.swpc.noaa.gov/products/solar-cycle-progression
Out of curiosity, I performed a simulation with KRAKsat orbit data. I gathered all TLEs from the beginning of its mission (or, to be more precise, from the 12th of July, ‘cause for the first few days, the TLEs were mistakenly swapped) and ran two scenarios in GMAT. One was a standard prediction of the orbit changes and one was set to start in July 2014 – 5 years earlier than the real mission started. Why 2014? As you can see in Fig. 7 this is the moment of a peak of solar activities – so its impact on the deorbit speed should be the greatest possible.
To be honest, simulation results really left me speechless. The difference is tremendous.
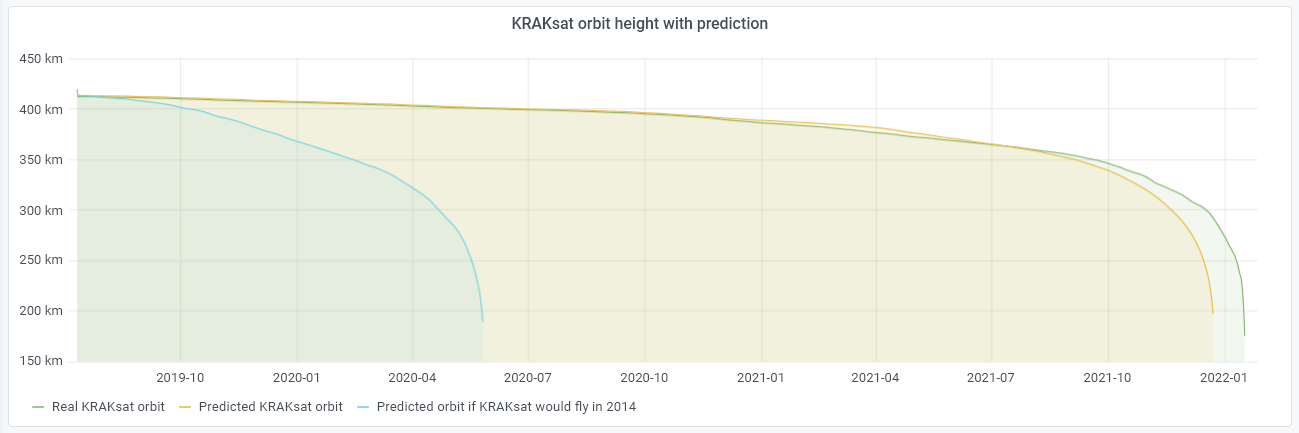
Fig. 8 - KRAKsat orbit height prediction for real case and for 2014 - LOOK AT THIS DIFFERENCE!
If KRAKsat was launched in July 2014, it would burn in the atmosphere in only 10 months! It would spend 3 times less time in orbit than it did in reality. Amazing, isn’t it?
While this is not a problematic case in student initiatives like this one (if KRAKsat worked properly, 10 months would probably be enough to perform the ferrofluid experiment), for bigger commercial projects this difference in mission duration could be extremely significant. Imagine an earth observation 6U CubeSat that has its operations planned for three years – a sudden shortening of this time threefold could simply cause its mission to fail by not performing all of its goals. And that is why choosing the right moment in time to start the mission on LEO (especially low LEO, ~400 km) is vital.
So, in short words, satellites fall because of the multiple forces that make them lose their total orbit energy. As a result, they fail to stick to their current orbit and, gradually, change their altitude. The more the orbit decays, the more height decreases – and the lower the altitude, the decay becomes faster and faster. Until the end.
How do we know when a satellite is going to decay?
When it comes to deorbit simulations I’d prefer not to go into details, because these are mainly mathematical calculations that are not very interesting in this kind of article. But in short: there are programs that calculate the so-called orbit propagation that is based on TLE, specific satellite parameters (mass, drag coefficient, cross-sectional area, etc.), atmosphere model, and space weather prediction. As a result, you can get information on how the orbit is going to change over time – and, of course, when (almost) exactly your satellite is going to reenter.
How precise are these calculations? It depends on the quality of data you put in :). For KRAKsat the simplest simulation forecasted that it’s going to decay on 22nd December 2021 – what happened, in reality, at night between 17th and 18th January 2022. In my opinion, this difference (less than a month in the context of a 30-month mission) is a negligible miscalculation (~3%). So pretty precise.
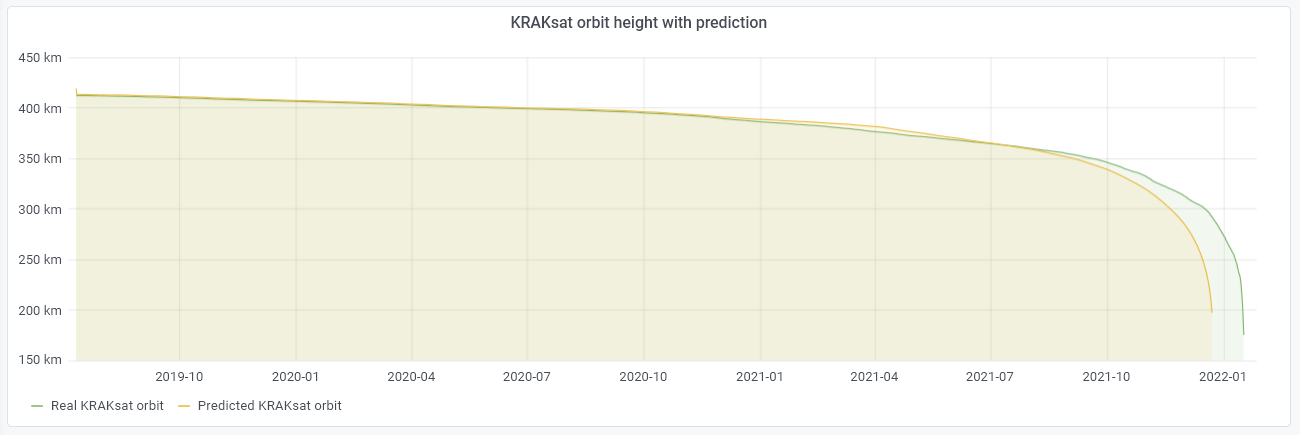
Fig. 9 - KRAKsat orbit height prediction and real reentry chart. Quite precise!
I used to work in GMAT (made by NASA! woho!) to compute these not very advanced simulations (with big help from Tomek who understands most of its intricacies). If you are interested in orbital mechanics and mathematics, have a look at this paper or some other publications on this topic. However, this is not trivial, so good luck :).
Why do small satellites burn during reentry?
As I wrote earlier, there are some parts of rockets or debris from bigger satellites that make their way back to Earth – but most CubeSats and other small spacecraft burn completely while entering the atmosphere. And this is not a result of sudden high temperatures somewhere on their way back home. In the last stages of reentry, the satellite traveling very fast encounters a more and more dense atmosphere. The heat from the friction of the air sets it on fire – and as it’s not designed to dissipate all of the heat, it’s just going to burn. According to the ESA website (Ref. 11), the satellite breaks-up finally at around 75 km.
❯ Note: At ~80 km there is the border between two layers of atmosphere – mesosphere and thermosphere. Temperatures in the mesosphere (45-80 km) are quite low, varying between 0 °C to −90 °C. But do you know what the temperature in the thermosphere (> 80 km) is? It can be as high as 2000 °C! You may then ask – why don’t all satellites burn while orbiting, huh? And this is a curiosity: an object in the thermosphere won’t experience the warmth, as the surrounding gas has extremely low density (almost vacuum) and the molecules simply cannot conduct this heat. If you place a normal thermometer there, you would read something significantly lower (Ref. 18). Interesting, isn’t it?
On another ESA website there is more information about reentry events:
Each day satellites, rocket stages or fragments thereof reenter the denser layers of the atmosphere, where they usually burn up. Shortly before reentry, at about 120 km altitude, spacecraft have speeds of typically 28 000 km/h. Only a few very large objects, such as heavy scientific satellites, reenter Earth’s atmosphere in a year. In total, about 75% of all the larger objects ever launched have already reentered. Objects of moderate size, i.e. 1 m or above, reenter about once a week, while on average two small tracked debris objects reenter per day.
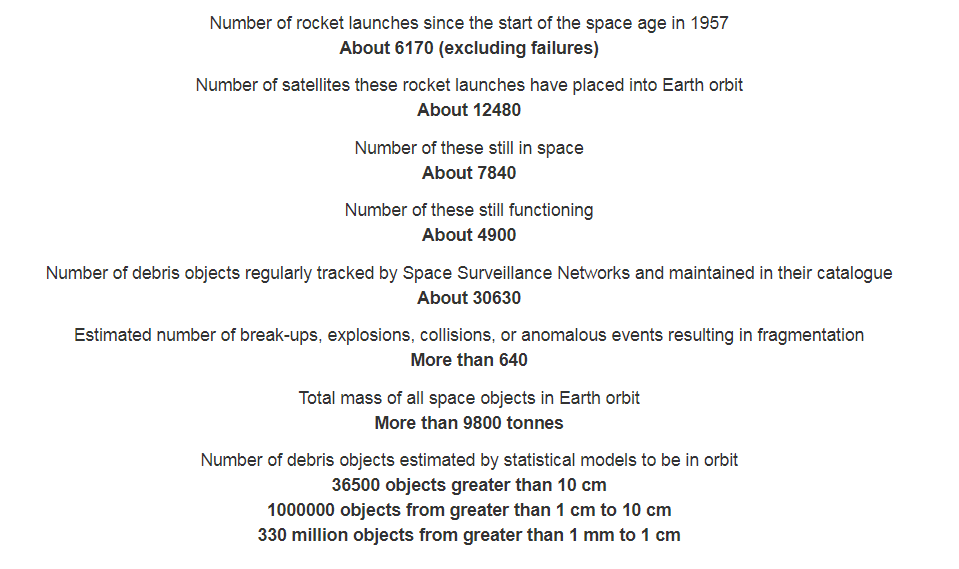
Fig. 10 - Statistics from ESA about space debris and reentries
Source: https://sdup.esoc.esa.int/discosweb/statistics/
There is even a map (Fig. 11) including recovered debris locations on land. Unfortunately, neither the image nor the article has a timestamp, so I have no idea how up-to-date this information is but still – interesting.
Usually, when it comes to small spacecraft, there’s almost no possibility to precisely calculate the time and place of the reentry before it happens. As the oceans cover about 70% of the Earth's surface, the chance is quite high that your cute cube is going to burn similarly to the small meteorite somewhere above the giant blue puddle. With no one to wave her goodbye…
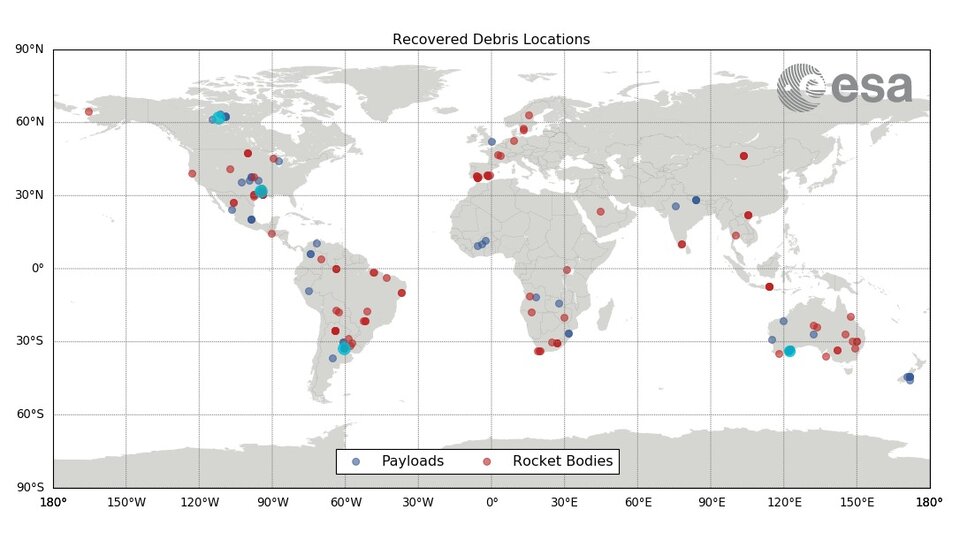
Fig. 11 - Map of recovered debris locations on land. A lot of falling trash!
Source: https://www.esa.int/Safety_Security/Space_Debris/Reentry_and_collision_avoidance
Deorbitation - regulations & statistics
How long can a satellite be in space? This is the tricky question because, as I wrote in an article about orbits, GEO satellites, placed 35786 kilometers from the Earth’s surface, aren’t really made to fall down – after their lifetime, they are placed in the graveyard orbit instead (although it’s worth to remember that they will eventually fall down, in hundreds of thousands of years). So it’s hard to talk about any limits, even with lower orbits (some debris from 60ties can still be found there!), but we can look at the… regulations.
There is something called The Inter-Agency Space Debris Coordination Committee which, as its name indicates, brings together space agencies from all over the world to develop legislation to reduce space debris. IADC Space Debris Mitigation Guidelines, containing the principles of orbit trash reduction, were first published in 2002 (with two revisions in 2007 and 2020). It says that every satellite launched into LEO or ending its operational phase in LEO should reenter in 25 years maximum (with a probability of 90%). Every other case should have separate handling, disposal plans, etc. What is crucial here is the word should. Unfortunately, these are only soft regulations, and IADC member agencies express their willingness to ensure that these regulations are complied with. Which is not the same as strictly obeying them.
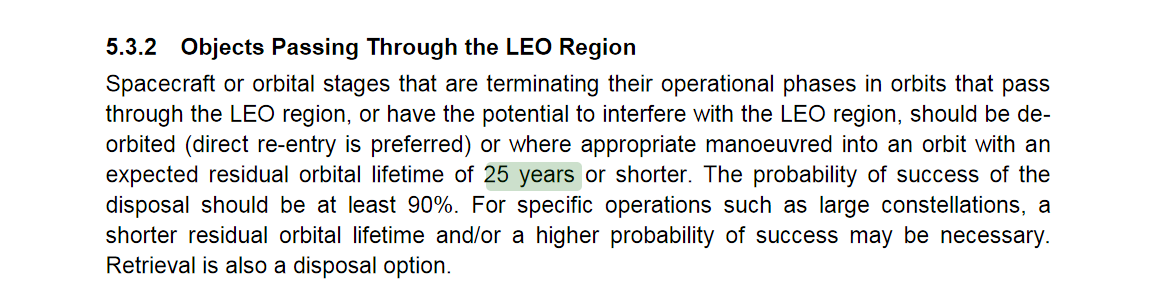
Fig. 12 - IADC Space Debris Mitigation Guidelines. Orbit would be a better place if everyone stick to these rules...
Source: https://orbitaldebris.jsc.nasa.gov/library/iadc-space-debris-guidelines-revision-2.pdf
These soft regulations highlight that any debris that survives reentry and could reach the surface of the Earth should not pose any risk to people and property. If this kind of thing is likely to happen, supervision and controlled reentry should be required as well as information to relevant air/maritime traffic.
There are many voices that debris guidelines should be tightened (Ref. 7), or, at least, their adherence should be monitored. But as it refers to the whole world, there is no such international tool that could allow prosecuting countries for breaking these kinds of laws.
Do we know how many organisations don’t follow the regulations? Yep. And this knowledge is saddening.
ESA runs a separate website called Space Debris User Portal that includes not only statistics but also interesting environment reports. The newest report points out that there are many satellite launchers who don't really care about the guidelines. According to ESA’s calculations, in the last decade, only 20-40% of payloads (excluding human spaceflight and these naturally compliant with law) attempted to deorbit in the 25 years. With rocket bodies, statistics look better as more than 40% performed a successful attempt to comply with the debris mitigation measures.
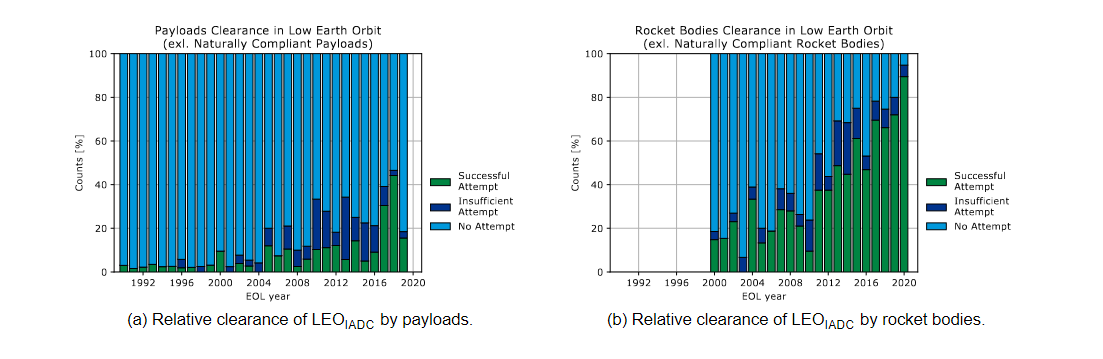
Fig. 13 - Statistics about the debris guidance compliance for LEO satellites & rocket bodies.
Source: https://www.sdo.esoc.esa.int/environment_report
As you can see, although the guidelines are explicitly defined, not everyone follows them – which in this case may be unfavorably noticeable only in the long-run.
The whole report is extremely absorbing, and I strongly recommend scrolling through the whole thing. And if you also would like to spy on deorbiting stuff on an everyday basis, there is an account on Twitter publishing information when something is going to reentry soon. They wrote about KRAKsat too 😭
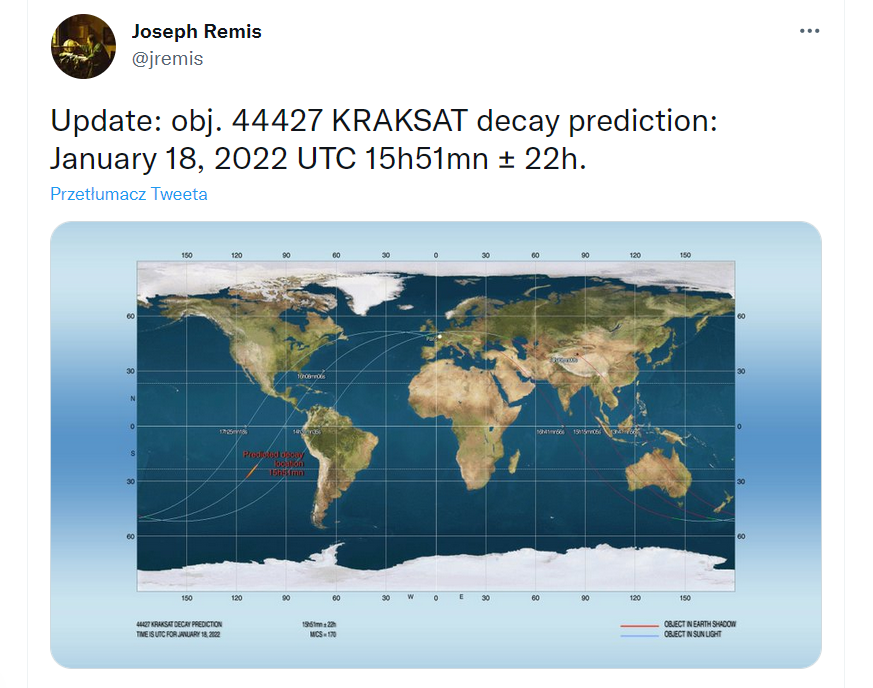
Fig. 14 - KRAKsat decay prediction. Unfortunately, it was quite right...
Source: https://twitter.com/jremis/status/1482280192596922370
How did the orbit parameters change during a satellite lifetime? KRAKsat case study
Do you remember the metaphor from the previous orbits article? Sit as you are sitting, close your eyes and quickly open them again – voilà! – if you were traveling with KRAKsat speed, you would be now sitting on the top of Mount Everest. Before you pass out from the lack of oxygen, let’s do a fleet calculation of what velocity is required to be able to stay still on the chosen orbit.
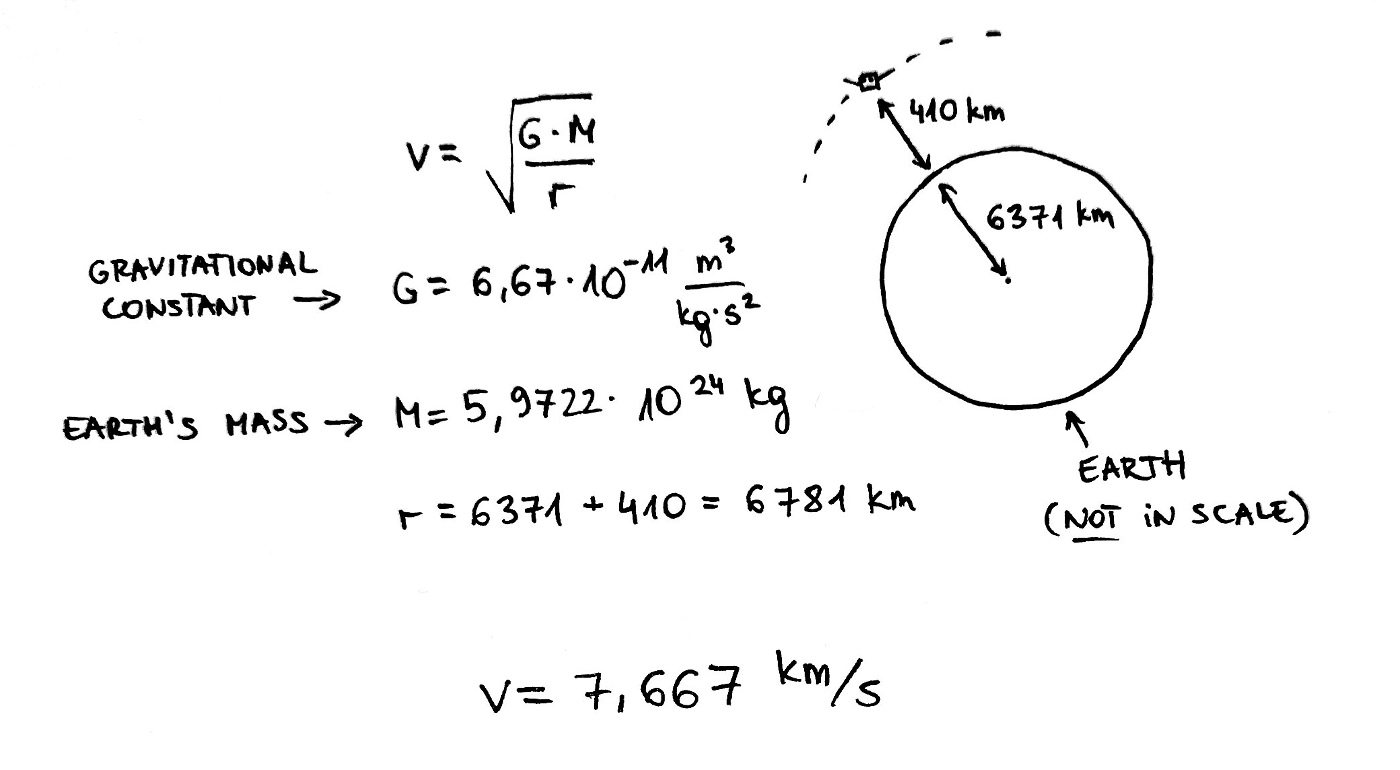
Fig. 15 - How to compute a satellite orbital speed?
We can see from the calculation that to be able to stay on 410 km (KRAKsat’s starting orbit), we would need to have our orbital speed equal to 7,667 km/s. As I already wrote, the atmospheric drag, solar activities, radiation pressure, and other factors cause the satellite to lose its energy – and, as result, to lower its orbit. And if we have a look at the change in KRAKsat’s velocity (Fig. 16)…
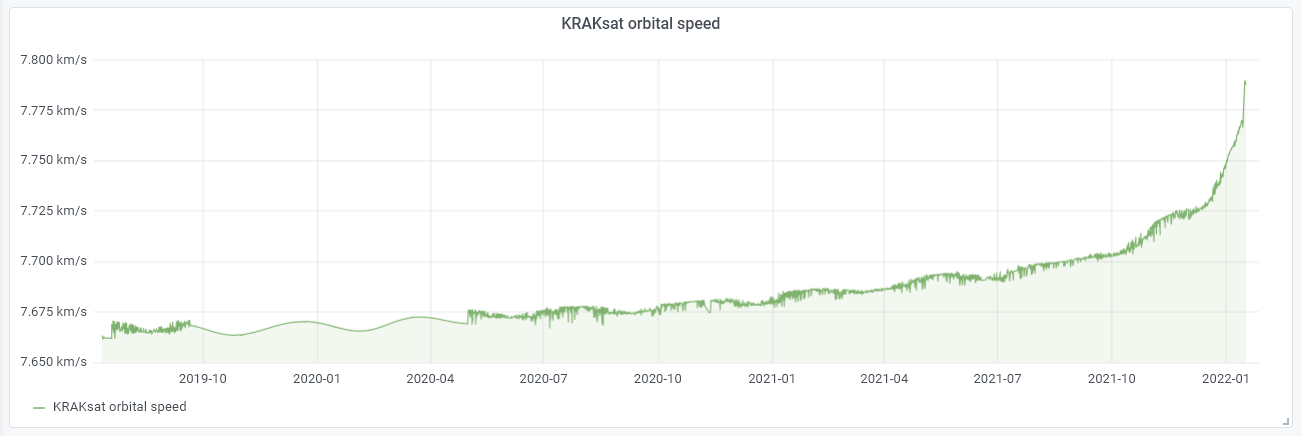
Fig. 16 - KRAKsat - orbital speed. Wohooo!
…we should see that the speed is going up. Why?
The topic is brilliantly described here. Even though there is a “negative” load on the system (effect of all disturbing forces), the change in spacecraft’s orbital speed is positive when it gets closer to Earth. It’s connected to the kinetic and potential energies – potential energy decreases with decay, so the kinetic one has to increase. And it happens all the time while the satellite is orbiting. This all may seem counterintuitive at first, but try to think about it for a moment – it makes sense!
We should also have a look at other charts. The parameter related to altitude is also the time of one orbit around the Earth. KRAKsat started with orbit length ~92 minutes, and it started to decrease in parallel to orbit height, dropping below 90 minutes somewhere near the last New Year’s Eve. It’s easy to understand – the cube decreased its orbit, so it simply took it less time to circle around our planet.
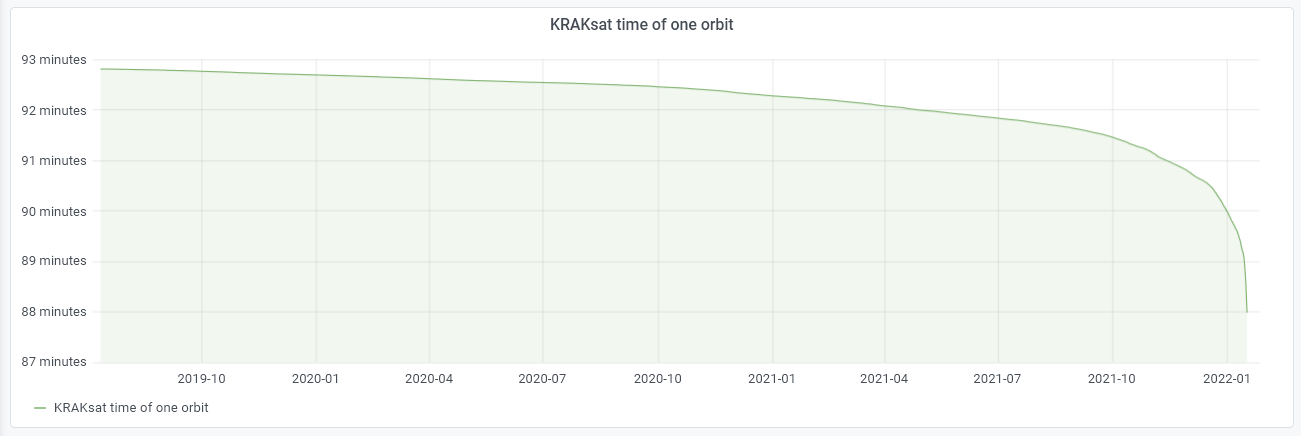
Fig. 17 - KRAKsat - time to perform one orbit. It also changed a bit!
In addition to height, inclination and eccentricity also shape a satellite’s orbit. And both of them changed significantly in the KRAKsat case – primarily at the end of its space life. Eccentricity got higher and more volatile, so the orbit stopped being close to a perfect circle, and the inclination decreased, making the angle between the equator and the orbital plane smaller.
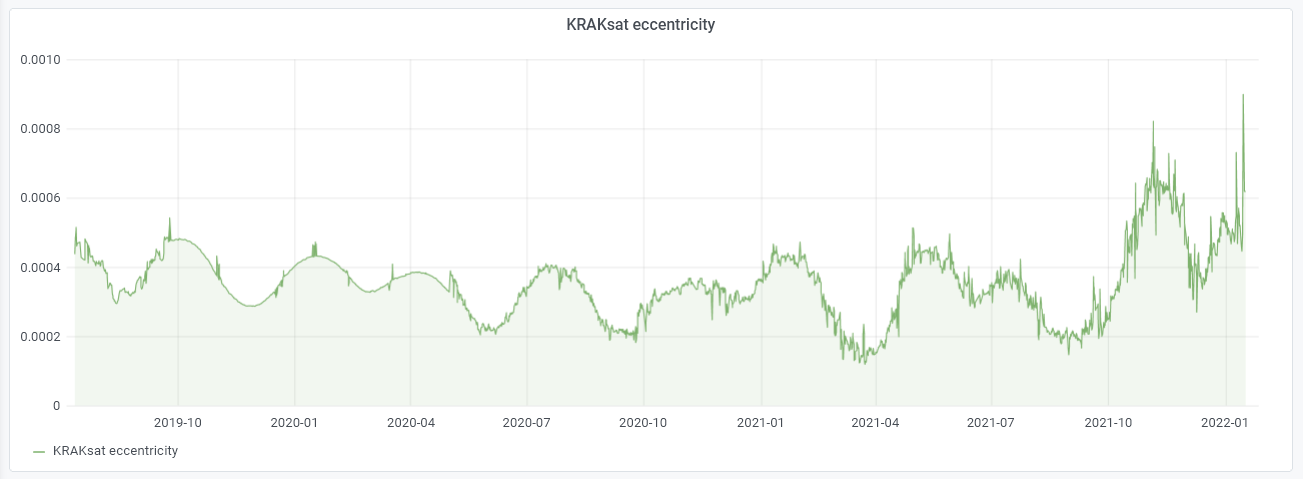
Fig. 18 - KRAKsat - eccentricity. Value of 0 would mean perfect circular orbit.
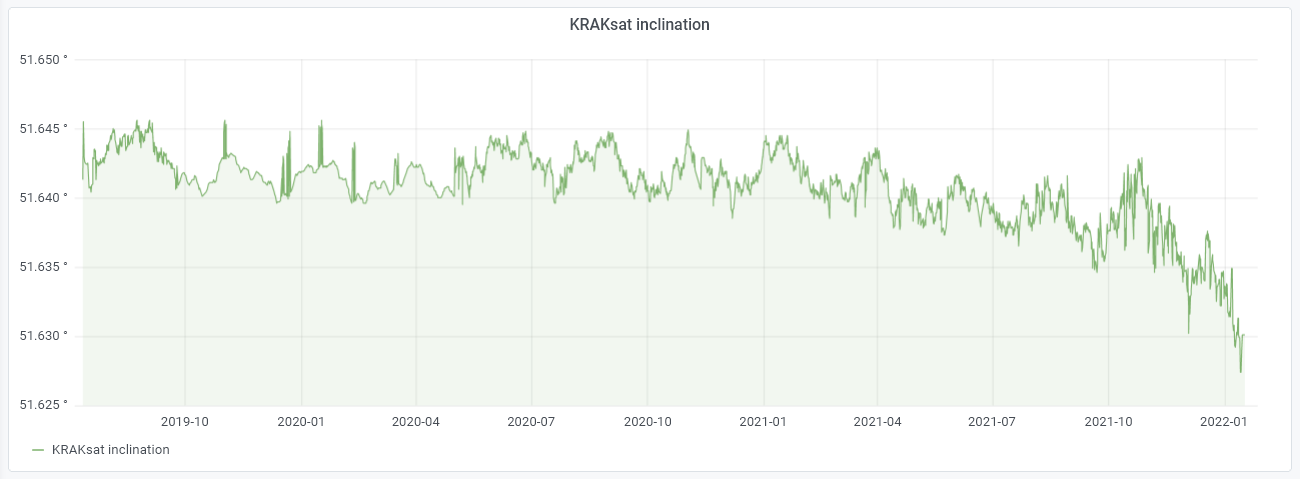
Fig. 19 - KRAKsat - inclination. Small changes, nothing significant.
When it comes to the computation of orbit parameters, there is one source of truth that is the most valuable: TLE. Without it, we would know almost nothing. For most of the time, in the KRAKsat case, it was updated quite regularly, twice a day. The flatten curves you can see in the graphs above (from October 2019 to April 2020) are there probably because of the fact that TLE was at that time updated less frequently. Until the 16th of January 2022, there were no problems with tracking or listening to our CubeSat – but on that day, the TLE was not revised. And it’s quite noticeable in the Fig. 20 below…
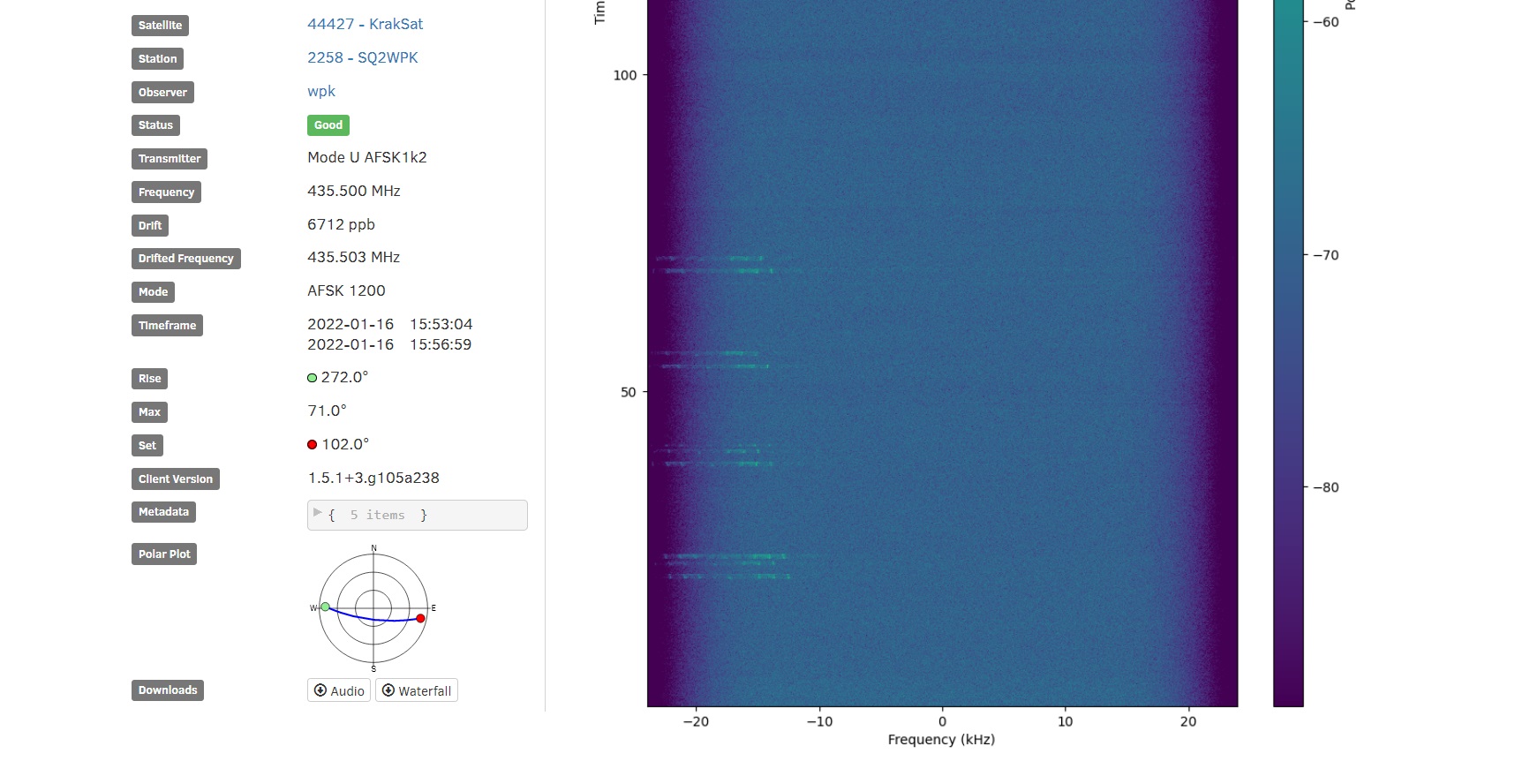
Fig. 20 - KRAKsat - observation from the 16th of January with old TLE. Signal should be centered and it's definitely not!
…that this lack of update became quite an issue. Changes in orbit were so remarkable, that it was near to impossible to decode anything automatically. Fortunately, there are many great people in the SatNOGS community and one of these guys helped me by computing the TLE from observations by himself (on the 16th January evening). Thanks to this, we were able to listen to KRAKsat during its last passes. As I already mentioned, the last stages of the satellite’s life happen very quickly, so not even a day had passed, and the cube became silent forever.
My last proper observation is from the 17th of January, 15:20 UTC. The final signal, probably a few hours before the reentry, was received by W2RTV at 19:39 UTC on the same day, and included BOOT NR 1713728. Yes, our crazy small cube restarted more than one million seven hundred thousand times.
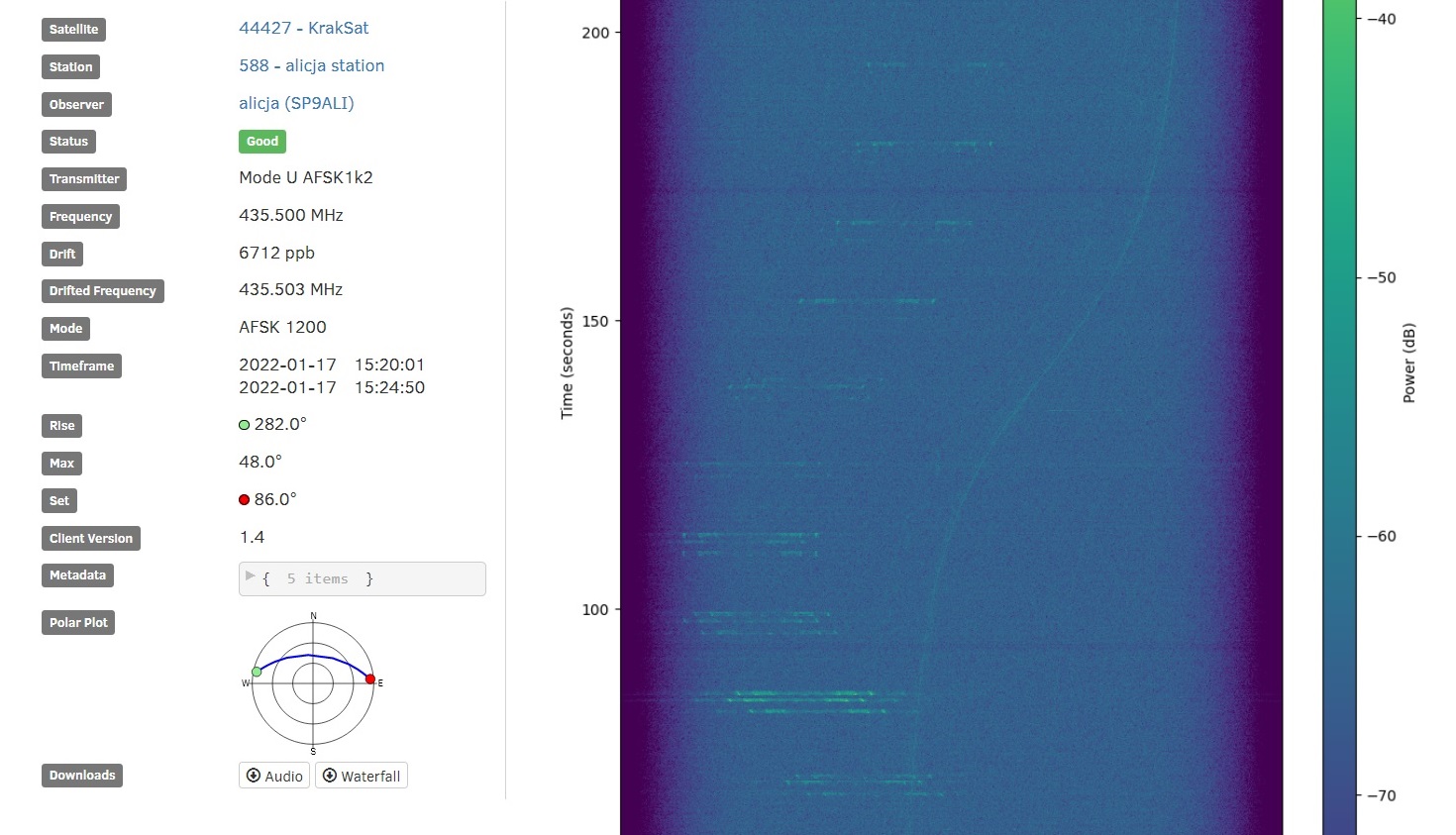
Fig. 21 - KRAKsat - my last observation - 17th of January, 15:20 UTC.
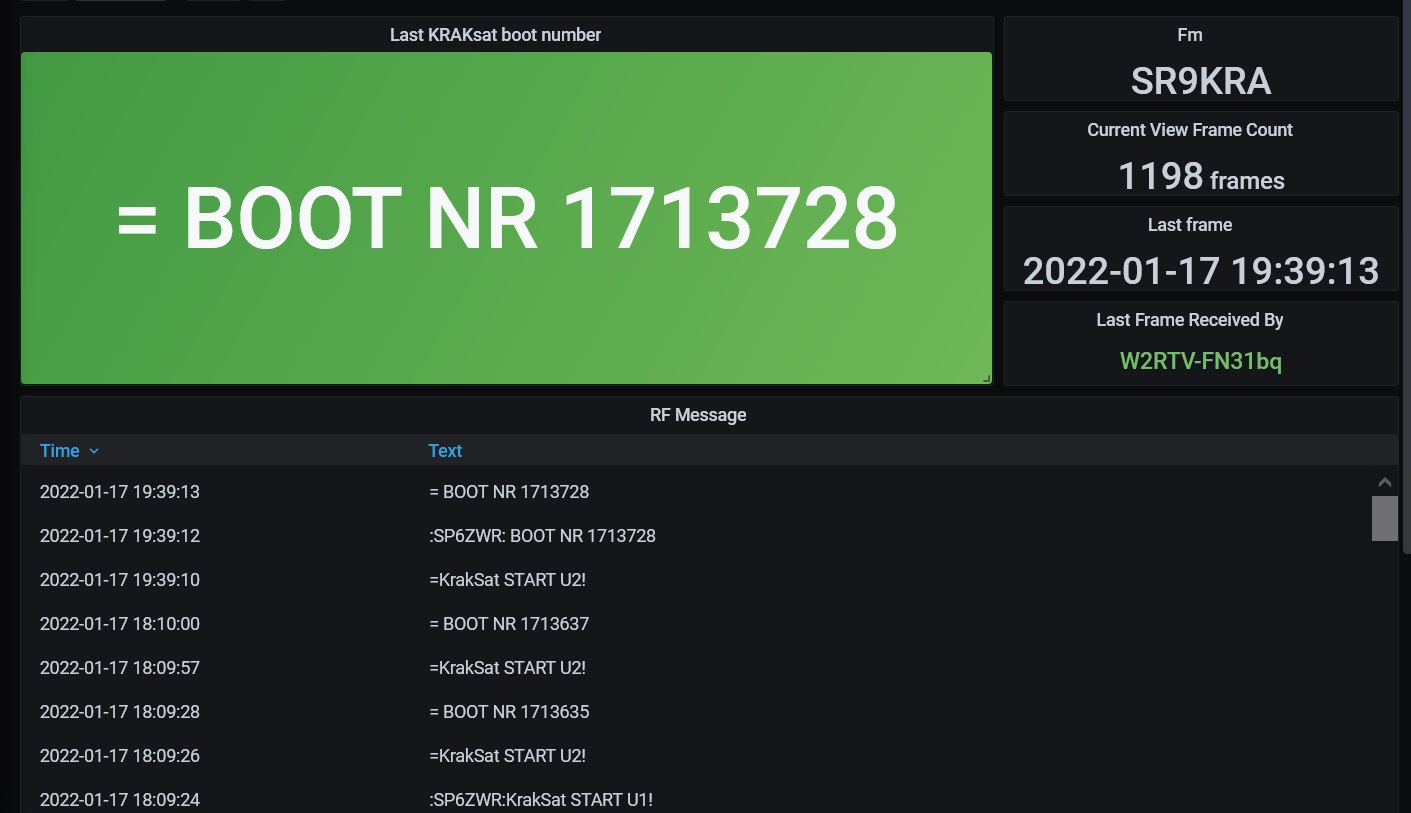
Fig. 22 - Last boot number decoded in SatNOGS
So Long, and Thanks for All the Fish
It was all a great adventure. I wanted to take this chance to say a big THANK YOU to all the people who were engaged in the project or helped us during this crazy space adventure. Thank you: KRAKsat team, PW-Sat2 & KP Labs teams, SQ6SKR, SQ5KTM, amazing radioamateurs from all over the world and SatNOGS community. It was a pleasure to meet you all and I have to admit that I am intimidated by how much support we got in all stages of this puzzling project.
❯ Fun fact: a few days before the decay, I managed to set up… a 25-meter radiotelescope to listen to it! These kinds of adventures only with SatNOGS :).
Goodbye KRAKsat, my small rebooting cube! You are going to have your place in my heart forever. I’m still extremely glad and thankful that I had the opportunity to be a part of this project. It all started by accident, and it’s still hard to believe what happened because of this in these few years.
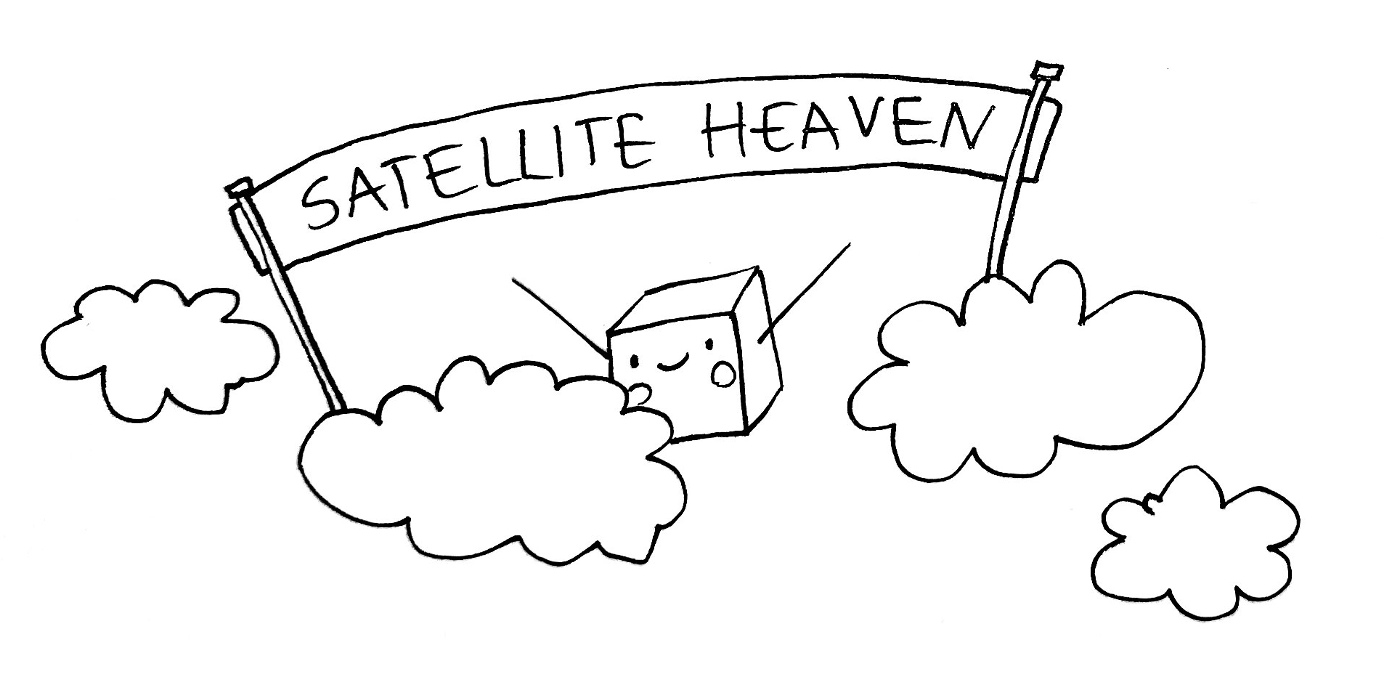
Now it’s time to focus on the forthcoming satellite projects that we are working on at KP Labs – if all goes well, our Intuition-1 will fly into space in about a year. Can’t wait!
References
- Satellite Orbits, January 2000, DOI: 10.1007/978-3-642-58351-3 Oliver Montenbruck, Eberhard GillEberhard Gill
- https://www.swpc.noaa.gov/products/solar-cycle-progression, [access: 18.01.2022]
- https://iopscience.iop.org/article/10.1088/1742-6596/1523/1/012010/pdf, [access: 18.01.2022]
- https://www.researchgate.net/publication/271729675_Impact_of_Solar_Flux_Modeling_on_Satellite_Lifetime_Predictions, [access: 18.01.2022]
- https://scialert.net/abstract/?doi=srj.2016.1.9 10.3923/srj.2016.1.9, [access: 18.01.2022]
- https://www.nasa.gov/smallsat-institute/sst-soa/deorbit-systems, [access: 18.01.2022]
- https://www.esa.int/Safety_Security/Space_Debris/Mitigating_space_debris_generation, [access: 18.01.2022]
- https://breakingdefense.com/2020/04/fcc-reconsiders-tightening-25-year-deadline-for-space-junk-disposal/, [access: 18.01.2022]
- https://orbitaldebris.jsc.nasa.gov/library/iadc-space-debris-guidelines-revision-2.pdf, [access: 18.01.2022]
- https://csps.aerospace.org/sites/default/files/2021-08/Reesman_SlashTheTrash_20200422.pdf, [access: 18.01.2022]
- https://www.esa.int/Safety_Security/Clean_Space/Space_debris_feel_the_burn, [access: 18.01.2022]
- https://twitter.com/jremis, [access: 18.01.2022]
- https://orbitaldebris.jsc.nasa.gov/library/iadc-space-debris-guidelines-revision-2.pdf, [access: 18.01.2022]
- https://amslaurea.unibo.it/19043/1/Thesis_DeCecio.pdf, [access: 18.01.2022]
- https://www.esa.int/Safety_Security/Space_Debris/Reentry_and_collision_avoidance, [access: 18.01.2022]
- https://sdup.esoc.esa.int/discosweb/statistics/, [access: 18.01.2022]
- https://earthobservatory.nasa.gov/features/OrbitsCatalog, [access: 18.01.2022]
- https://spaceplace.nasa.gov/thermosphere/en/, [access: 18.01.2022]
- https://conference.sdo.esoc.esa.int/proceedings/sdc8/paper/297/SDC8-paper297.pdf, [access: 18.01.2022]
- https://www.wired.com/2010/11/changing-orbits-and-changing-speed/, [access: 18.01.2022]
- https://en.wikipedia.org/wiki/Orbital_decay, [access: 18.01.2022]
- https://courses.lumenlearning.com/suny-osuniversityphysics/chapter/13-3-gravitational-potential-energy-and-total-energy/, [access: 18.01.2022]
- https://courses.lumenlearning.com/suny-osuniversityphysics/chapter/13-4-satellite-orbits-and-energy/, [access: 18.01.2022]
- https://www.saburchill.com/physics/chapters/0010f.html, [access: 18.01.2022]